Orion, the showpiece constellation of winter, and probably the most-recognized constellation. This beautiful constellation is rich with interesting objects, science, and mythology, and is a useful signpost to a large number of other interesting objects.
Finding Orion
From late fall to late winter, Orion appears in the South East sky, moving through the South, to the South-West. It is always a comfortable distance above the horizon usually at an altitude (from Ottawa) of 30 to 40 degrees. So, just look toward the south, slightly up from ground level (less than halfway to “straight up”), and look for the familiar shape. Orion in late November, 11:00 PM
Table of Contents
- Finding Orion
- Orion the Hunter
- Orion’s Mythology
- Orion with Celestial Equator
- Nebulae
- Finder chart for M42
- Orionids
Orion the Hunter
Orion is one of the rare constellations that actually look somewhat like their mythological character in this case a hunter, with a tight belt, wearing a sword and brandishing a club and a shield.
Look for the distinctive “belt” first. These are closely- and evenly- spaced bright stars in a straight line. From the belt, you can find the 4 corners of the body (shoulders and knees, or shoulders and feet, depending on the interpretation). In darker skies, you will also be able to see the 3-star “sword” hanging from the belt. The hunter’s raised club and extended shield are dimmer stars, and require dark skies outside the city light pollution to see.
Orion is not always “vertical”, but is rarely far from it. He is a large constellation, stretching about 17 degrees in the sky from foot to shoulder (just under the width of two clenched fists held at arms length).
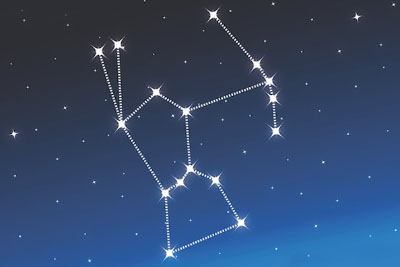
Orion’s Mythology
Orion was the son of the god of the sea (Poseidon to the Greeks, Neptune to the Romans). He loved the godess of animals and the moon (Artemis to the Greeks, Diana to the Romans), sister of Apollo. Apollo did not approve.
He was a great hunter, and is located in the sky standing near the river Eridanus, accompanied by his two hunting dogs Canis Major and Canis Minor. He is bradishing his club, and holding his outstretched shield, while fighting nearby Taurus, the bull.
Orion is usually considered related in some way to Scorpius, the giant scorpion, because they are close to 180 degrees apart in the sky, so one is always setting exactly when the other is rising. Myths either say Orion is hunting the scorpion or running from it.
In his youth, Orion was rendered blind by King Oenopion, for chasing his daughter Merope (one of the Pleiades, the Seven Sisters), but his sight was restored by the rising sun.
There are several versions of the story of Orion’s death. Orion is of interest to amateur astronomers for many reasons.
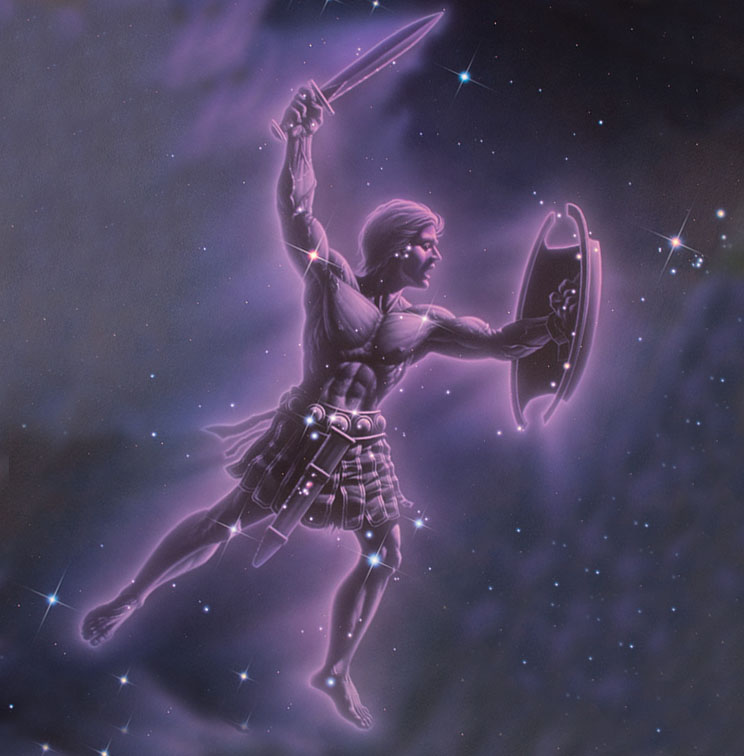
Orion with Celestial Equator
First, he is a useful reference for declination. Mintaka, the rightmost star in Orion’s belt, is at almost exactly zero declination, which means the belt is a useful reference for Declination 0, the Celestial Equator (the projection of the Earth’s equator into the sky.) So, to people on the equator, Orion will pass directly overhead, straight up.
Stars
Orion contains many interesting stars. The two most prominent are Rigel and Betelgeuse.
Rigel
Rigel, the bottom right star, is of interest for being one of the brightest stars in the sky. (It is ranked 6th not including the Sun; between Vega and Procyon.) It is a Blue-White Giant, with visual magnitude 0.1, and is about 55,000 times more luminous than our Sun.
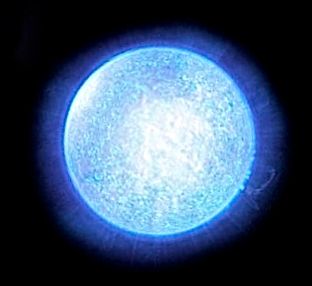
Betelgeuse
Betelgeuse (“beetle juice”), the noticeably red top left star in the main body (the “shoulder” on our left), is an irregularly pulsating Red Supergiant. Its size varies from 300 to 600 times the diameter of our Sun. (If it replaced our Sun, Mercury, Venus, Earth, Mars, and Jupiter would be inside the star.)
Betelgeuse is so large that in 1996 it became the first star to have its actual disk imaged from Earth by the Hubble Space Telescope.
Our own Sun will become a Red Giant in a few billion years, although it will be smaller than Betelgeuse only about 200 times its current size.
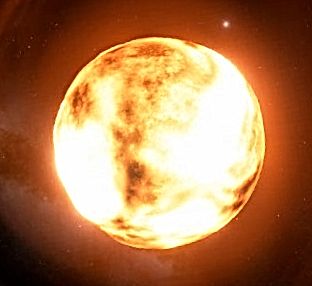
Nebulae
What Orion is best known for, however, is its collection of nebulae.
The entire Orion region is engulfed in a huge cloud of Hydrogen the Orion Molecular Cloud Complex. This cloud is about 1500 light-years away from us, and is hundreds of light-years across. The entire cloud can be seen in wide-field, long exposure photographs of the region.
Several denser components of the cloud have their own names and are within reach of small telescopes.
M42, the Great Orion Nebula, and nearby M43, are probably the most-viewed and most-photographed nebulae in the entire sky. This cloud is a stellar nursery, and Hubble Space Telescope images confirm that we can see dozens of new stars in the process of forming.
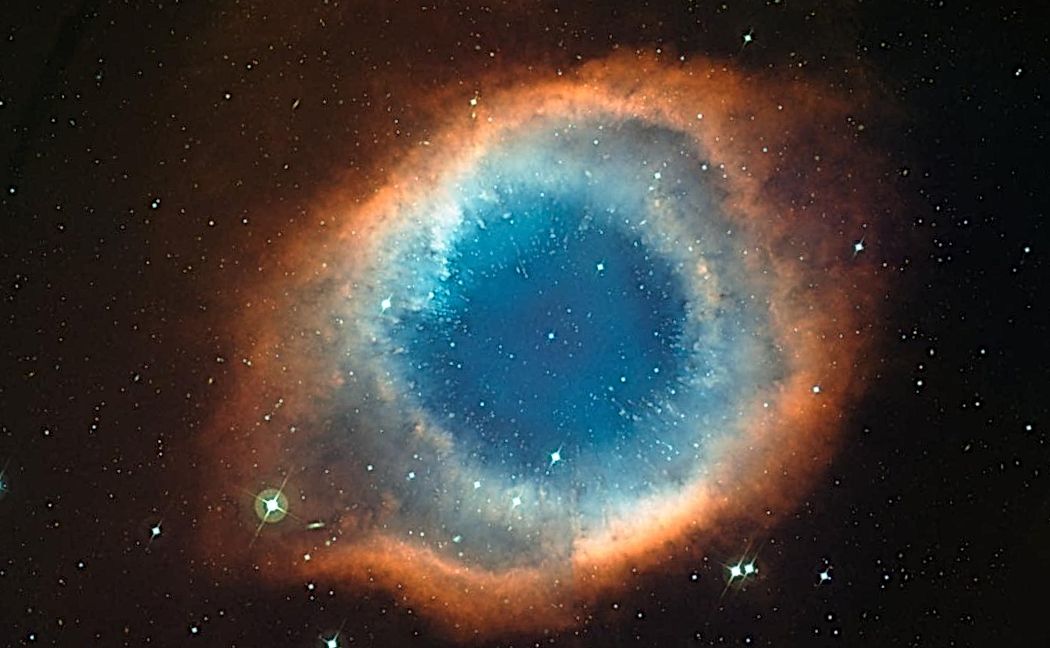
Simulation of the appearance of M42 in a small telescopeM42 is so large and so bright that it is visible to the naked eye in dark skies, in ordinary binoculars, and in even the smallest telescopes. However, you should be aware that a small telescope and eyepiece will not show you the spectacular colours you have seen in photographs. At the eyepiece, nebulae appear in shades of gray.
Finder chart for M42
M42 could not be much easier to find point your telescope or binoculars at the middle star of Orion’s sword
In a telescope, you will also see that the “star” in the centre of the nebula is actually a multiple star system, with at least 4 stars visible to small scopes.
Amateurs with large aperture telescopes (over 10 inches) and very dark skies, or any amateurs with long-exposure astrophotographic capability, can also see the beautiful Flame Nebula (NGC2024, next to Alnitak, the left star in Orion’s belt), and nearby IC434. Flame Nebula
Next door to the Flame Nebula, IC434 is most famous not for its own sake, but because of a blob of dust that sits in front of it, creating a dark region. This “dark nebula”, Barnard 33 in the Barnard catalogue of dark nebulae, is called the “Horse Head Nebula” for obvious reasons. Note that the Horse Head is small and very difficult to see. It is a difficult challenge object for the visual observer, requiring large aperture and a narrowband filter.
Orionids
Orion is also the point of origin for the Orionids meteor shower, which occurs annually in the third week of October. While this is not the most spectacular of the year’s meteor showers, it is interesting for its origin it results from the Earth passing through the dusty remnants of the tail of the passing Halley’s Comet.