In the 1940’s and ‘fifties a handfull of brilliant, massive O and B stars were discovered which exhibited space velocities higher than normal for such youthful members of the Galactic disk. Interstingly, a few of them had proper motions whose vectors pointed directly away from the then known young association of O and B stars in central Orion. Furthermore, the theoretical ages of the association and the presumably escaping former members were in reasonably good agreement. Clearly some event or events kicked these stars out of the nest, so to speak. To differentiate these young fast movers from the ancient high velocity stars, a new term suggested itself: runaway stars.
During the decades following, more O and B runaway stars were found in other parts of the sky, and in many cases a young stellar group could be identified as a likely parent for each. How is it we can be at all certain when establishing such links?
Table of Contents
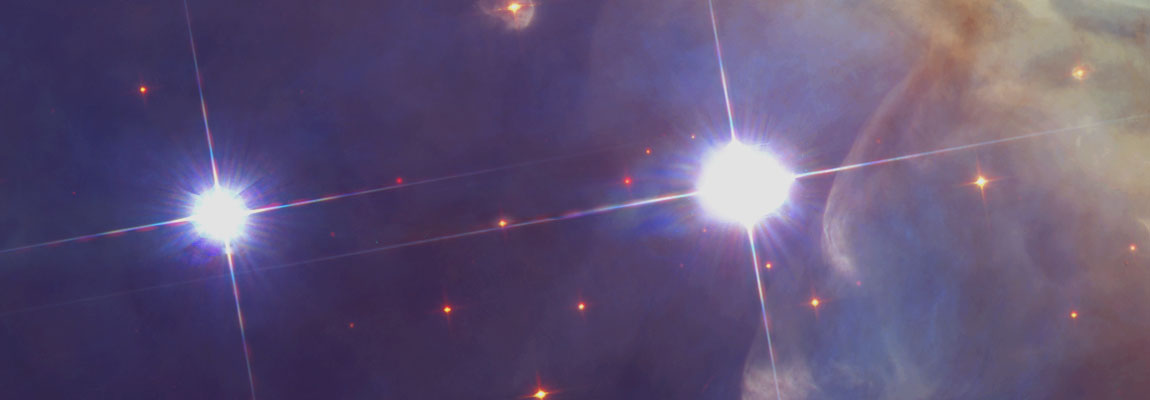
The gas clouds from which disk stars are born follow very nearly circular orbits close to the Galactic disk’s midplane, and so they and their progeny have small velocity differences, typically 7-10 km/s. The most massive newborn stars are of spectral types O and B, and live short lives of no more than a few tens of millions of years before exploding as supernovae. Therefore under normal circumstances such a star cannot be found very far from its birthplace. If a star were to be ejected, for any given velocity we can determine a maximum distance it could travel based on its expected life span, and in most cases this will not be so far as to make its origin too ambiguous. Of course, the foregoing presupposes that the distances and space velocities for the star and any candidate parents have been determined to sufficient accuracy!
Incidentally, when astronomers conduct searches for runaways from stellar catalogs they might initially choose as a lower limit a velocity of three times the 1-sigma value of the velocity dispersion for the stellar population under consideration. For OB stars the 1-sigma velocity dispersion is 10 km/s, so three times this is 30 km/s. From statistics, a Gaussian distribution has 68% of the population within 1-sigma, 95% within 2-sigma and 99% within 3-sigma. So only 1% of a population will be found outside of the 3-sigma limit, for which the term anomalous might be a fair characterization. Of course, some true runaways can have peculiar velocities of less than 30 km/s, as has been amply borne out in reality.
It’s worth pointing out that not only massive stars can become runaways. Indeed, because lightweight stars are so very much more numerous, one would expect that there should be a correspondingly larger number of them as runaways. But their dimness makes their detection possible only if they lie rather close to us. Possibly a very small fraction of the low-mass high velocity stars are young runaways. But let’s restrict ourselves to the massive, easily detected variety.
The List of Runaway Stars
For each star, the data are listed in the following order: name; visual magnitude; full M-K spectral type; mass in solar units; distannce in light years; and velocity, in kilometers per second, with respect to the local standard of rest (LSR) at the location of the runaway. For binary stars the magnitude and mass are for the pair combined. Many distances are somewhat uncertain, especially for the more distant objects. In a few cases I had to calculate a distance based on the available trigonometric parallax and spectral type (spectroscopic parallax).
AE Aurigae, Mu Colombae and Iota Orionis
A dynamical ejection from the Trapezium cluster 2.5 Myr ago
AE Aur 6.0m O9.5V 16 solar 1450 l-y 113 km/s
Mu Col 5.2m O9.5V 16 solar 2000 l-y 108 km/s
Iota Ori 2.8m O9III+B1III 38 solar 1450 l-y 8 km/s
This trio of runaways could rank as the most interesting of their kind in the entire sky. The evidence indicates that these four stars once comprised a quadruple system within the Trapezium cluster. Out of the complex gravitational dance they were engaged in, two singletons were ejected at high velocity, while the heavier binary (Iota Ori) was kicked out of the cluster at a more leisurely pace. Imagine if these stars were today still located within the Trapezium!
Like some other runaways, AE Aur is by chance passing through an unrelated interstellar cloud and is lighting it up as the emmission/reflection nebula IC 405, the Flaming Star nebula.
53 Arietis A binary supernova ejection from the OriOB1a association 4.3 Myr ago?
53 Ari 6.1m B1.5V 10 solar 1650 l-y 39 km/s
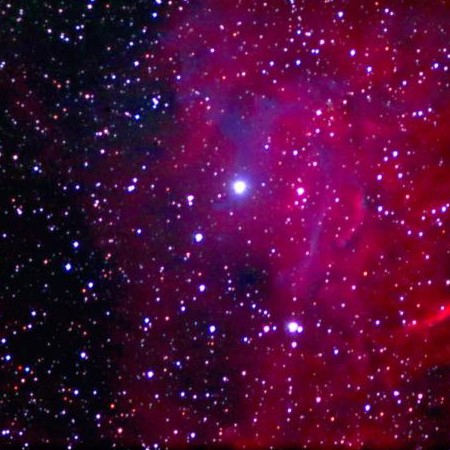
The origin, age and ejection mechanism for 53 Ari is still uncertain. It has almost certainly departed the Ori OB1 association, but which sub-group? Ori OB1c and d would seem to be too young, but sub-group b could be old enough to have ejected 53 Ari dynamically. To clinch the issue further evidence will be required.
Geminga (neutron star)
A binary supernova ejection from the Lambda Ori cluster 0.35 Myr ago
HIP22061 7.2m B2.5V 8 solar 2100 l-y 87 km/s
HIP29678 5.9m B1V 12 solar 2150 l-y 63 km/s
Geminga 1.4 solar 2000 l-y? 300 km/s?
HIP22061 and HIP29678 form an analogue of AE Aur and Mu Col–two similar-mass stars flung in opposite directions at similar velocities (it’s surprising the latter, at 5.9m, did not receive a Bayer number). The re-traced orbits of these two stars do not coincide exactly with the cluster, but an as yet undiscovered third party could resolve the discrepancy.
Also flying away from Lambda Ori for the past 350,000 years is the neutron star Geminga. No, you’ll never see this one! However it’s interesting in that it’s the most likely cause of the blasted out seven degree wide bubble and ring of molecular clouds surrounding Lambda. The ring includes the dark clouds B30, B31, B35, B36, B224 and B225, and the bubble itself is the very faint emission nebula Ced 54 (Sh2-264).
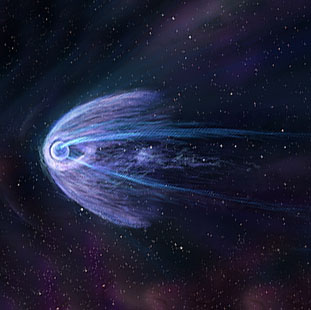
Xi Persei
A binary supernova ejection from the Per OB2 association 1 Myr ago
Xi Per 4.0m 07.5III 34 solar 1190 l-y 65 km/s
The runaway nature of Xi Per is revealed only by its large radial velocity, directed almost exactly away from us. Therefore Xi is on the far side of Per OB2, about 150 l-y beyond. The cloud Xi is illuminating as emission nebula NGC1499 (the California nebula) is not so distant from Per OB2 as to be unrelated
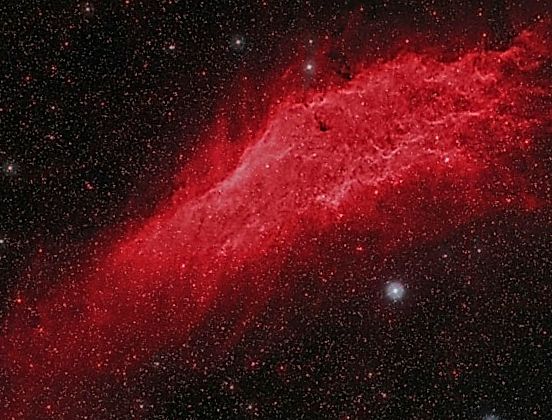
Alpha Camelopardalis
A binary supernova ejection from the Cam OB1 association ~4 Myr ago?
Alpha Cam 4.3m O9.5Ia 30 solar 2600 l-y 20 km/s?
The proper motion of Alpha Cam (also called Lucida) is directed more or less away from the Cam OB1 association. The distance of these objects is too far for the Hipparcos parallaxes to be of much help, and there remains some uncertainty regarding the particulars of both.
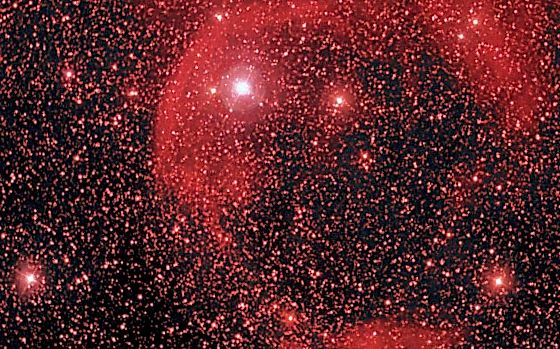
Nu Andromedae
A dynamical ejection from the Lac OB1 association 9 Myr ago
Nu And 4.5m B5V+F8V 7 solar 435 l-y 32 km/s
This star is often used as a locator for M31. During the 9 Myr since its leaving Lac OB1, the association has also traversed a considerable distance. That’s why the reciprocal of Nu’s proper motion vector points back so far away from its parent. Moreover, Nu is considerably closer to us–Lac OB1 is 1,200 l-y distant
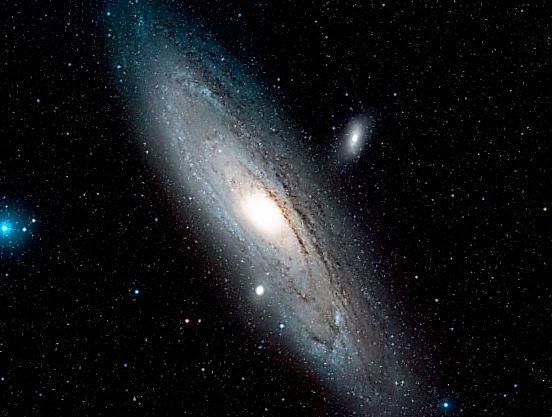
Lambda Cephei
A binary supernova ejection from the Cep OB3a association ~4.5 Myr ago
Lambda Cep 5.1m O6I 40 solar 1500 l-y 74 km/s
Lying as it does near the Galactic plane, it’s not surprising that Lambda is dimmed due to interstellar dust by 1.6 magnitudes, or a little more than a factor of four. Much of its space velocity is directed toward us, and over the past 4.5 Myr would have traveled nearly 1,100 l-y (Cep OB3 is about 2,400 l-y distant). Cep OB3a is the older sub-group (7.5 Myr vs 5.5 Myr for subgroup b), and is the more likely parent of the star which subsequently exploded after some 3 Myr. About 2.5 Myr ago Lambda passed fairly close to the association Cep OB2, and is currently about 500 l-y closer to us than the latter.
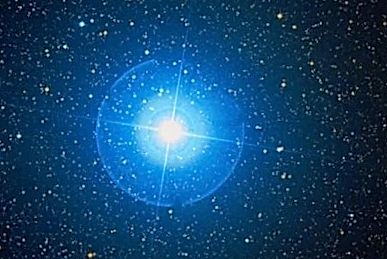
68 Cygni
An ejection from the Cyg OB7 association?
68 Cyg 5.0m O8 23 solar 2500 l-y km/s
Of the runaway stars presented here, this is the one about which least is known by me at the time of writing. The insignificant Hipparcos parallax and the lack of a spectral luminosity class result in an uncertain distance that can lie anywhere between 1,600 and 4,000 l-y or more. Taking into account the dimming of 0.75m (a factor of 2) by interstellar dust, and assuming the star is still on or near the main-sequence, a distance of about 2500 l-y is obtained. This is close to the ~3,000 l-y determined for the loose (and somewhat doubtful) Cyg OB7 association, and the star’s proper motion vector is carrying it away from the vicinity of that association.
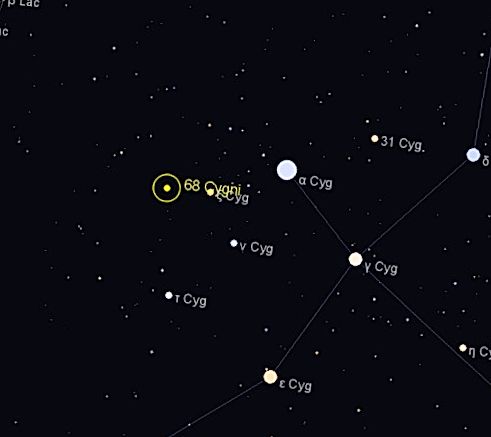
As of this writing I have no radial velocity information on hand which would allow a determination of the total space velocity, but the proper motion at the assumed distance of 2,500 l-y yields a heliocentric tangential velocity of 35 km/s (this is with respect to the Sun, and not to 68 Cyg’s LSR).
Like some other runaways, 68 Cyg is currently passing through an interstellar cloud and is lighting it up as the very faint, 2.5 degree long emission nebula Sh2-119.
Zeta Ophiuchi and PSR J1932+1059
A binary supernova ejection from the Sco OB2 association 1 Myr ago
Zeta Oph 2.5m O9.5V 16 solar 455 l-y 24 km/s
PSR J1932… 1.4 solar 800 l-y 240 km/s
Here is a rare case where a runaway compact remnant of a supernova has had its origin reasonably firmly established. The pulsar’s quite high space velocity is almost certainly due in no small measure to the kick it received during the explosion. The initial velocity was likely about 350 km/s, but the gravitational pull of Zeta Oph would have decelerated it to its present speed. Zeta is lighting up its environs, the emission nebula being the very faint, seven degree diameter Sh2-27.
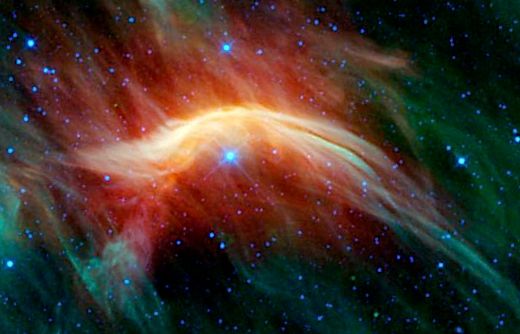
High Velocity Stars
About one hundred years ago it was realised that some stars travel through space at rather high speeds, in some cases 200 km/s or more. Naturally, a term was coined to describe these seemingly anomalous objects: high velocity stars.
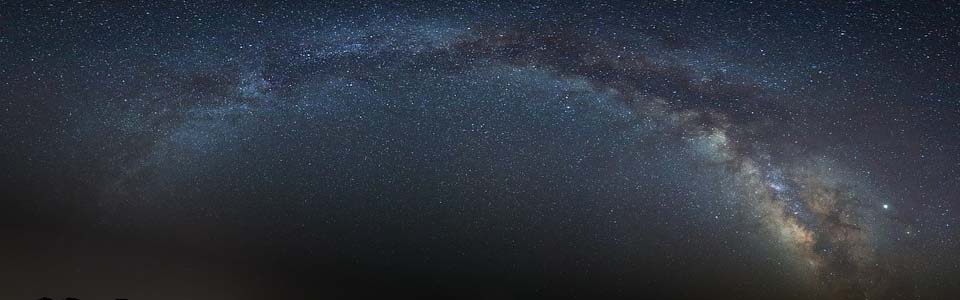
Today we’re pretty certain we understand the nature of the high velocity stars. With a knowledge of the “shape” of the gravitational potential of the Galaxy, orbit re-tracing reveals that the great majority of high velocity stars are visiting us from the inner parts of the Galaxy. In other words, they’re on elongated orbits—in many cases highly inclined—which carry them from the more densely populated Galactic bulge out into the solar neighborhood. And being currently on the outer parts of their orbits, they’re traveling more slowly. This, coupled with the often significantly different (noncircular) direction of motion, results in these stars actually lagging behind the disk stars (of which our Sun is one). The velocity differences as seen from our moving perspective makes them appear to be whizzing past us, whereas it is us and the other disk stars who are speeding ahead.
As more high velocity stars were uncovered, it was found that they’re almost exclusively low mass main-sequence dwarfs (e.g., Barnard’s star) or intermediate mass giants (e.g., Arcturus). And their spectrograms reveal a smaller heavy element content than Sunlike stars. These facts provide further confirmation of their origin, namely the 10-13 billion year old bulge/halo population which includes some of the oldest stars of the Galaxy and whose more massive bretheren have long since disappeared from the scene.
Dynamical Ejection
Immediately after formation, a star cluster or unbound association contains its greatest number of stars, generally in a more compact configuration. While this leads to more opportunities for interactions between stars, the small internal velocity differences of 1-5 km/s in a cluster or association can not easily create runaways. But a far more efficient “engine” is at hand: binary stars.
At least 50% of all stars are involved in binary or multiple systems. This is no different in young stellar groups, and youth means there will be some systems which contain massive stars. A binary whose members orbit each other sufficiently closely is called a hard binary, and can not easily be disrupted. If the stars are heavyweights they will orbit the common centre of mass at speeds of (typically) 30-150 km/s—much faster than the aforementioned velocity dispersion of 1- 5 km/s.
Any star venturing near a hard binary can be significantly accelerated due to the transfer of angular momentum. Usually the interloper will be temporarily captured, thus forming a hierarchical trinary with either it or one of the binary members going into a highly eccentric orbit. At each close approach, or periastron, of said star an exchange may occur, where it and one of the stars of the closer pair will effectively swap places, with the large, eccentric orbit becoming much smaller and vice versa. Eventually, after 10 – 100 such exchanges, one of the three will be ejected at a speed comparable to the orbital velocity—a runaway is born.
Of course, two binaries may interact, resulting in possibly as many as four runaways, but more usually two. It is this very situation which is the most likely cause of the runaways AE Aur, mu Col and the iota Ori binary.
Supernova
Every now and again, you hear of an amateur discovering something completely unexpected: McNeil’s Nebula comes to mind. It’s the result of great luck coupled with the skill and experience to recognize that luck.
Supernova hunting is another amateur-dominated activity. Again, barring luck, the process is driven by perseverance. It does take a good deal more equipment than comet hunting, but amateurs can make a lot of solid contributions.
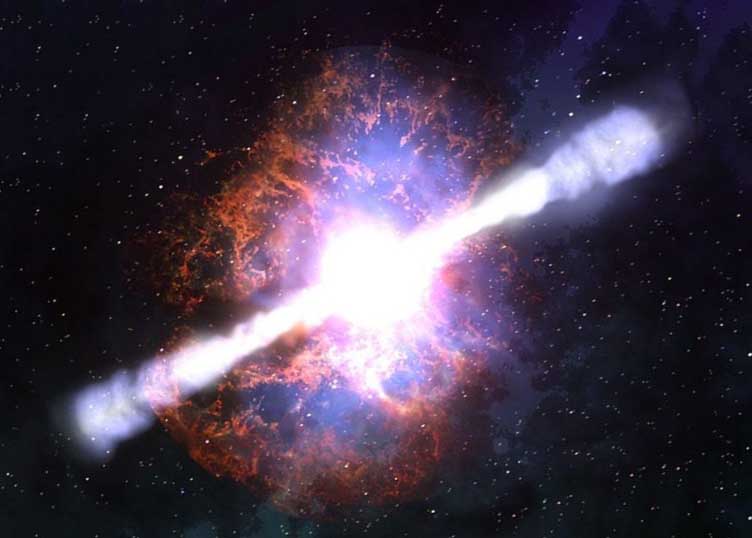
Hunting for extra-solar planets is a current hot area, and it’s been the exclusive preserve of professionals. Then, in late August, a rather obscure news item described the discovery of an extra-solar planet orbiting a faint star in Lyra. Here’s the real gem of the story it was discovered with a 4-inch telescope built in a garage. The Trans-Atlantic Exoplanet Survey is run by professionals, who’ve spent years writing software and tuning their gear. Their expertise and training are absolutely essential to the task. But they use off-the-shelf equipment and their ingenious analysis software is working.
Geniuses and professionals are blazing the trail, but lesser mortals can follow. Even now, amateurs can measure the light-curves of exoplanet transits. How much longer will it be before high-end amateurs make their first discoveries?
When we eventually find our first Earth-like exoplanet, it probably won’t be the work of an amateur. But I’m willing to predict that soon after that first discovery, amateurs will be observing the transits of those small, rocky worlds.
Binary Supernova
Stars weighing about 10 solar masses or more will eventually explode as supernovae. If a binary is comprised of such a star and a companion in a sufficiently close orbit, subsequent evolution of the system can result in a runaway star. Let’s consider the case where the stars are close enough so that mass transfer can take place. Furthermore, let’s assume the two stars are not too dissimilar and are on more or less circular orbits.
As the more massive star evolves it will swell up to become a supergiant first. When its outer envelope completely fills the star’s Roche lobe, material will flow through the point where the two stars’ Roche lobes meet and then spiral down onto the companion. This matter will most likely be enriched with helium synthesized within the supergiant. Moreover, this infalling gas will increase the companion’s rotation speed as it crashes onto the surface. So the companion will tend to become a helium-rich rapid rotator, as well as a somewhat brighter blue straggler because of the extra fuel it received.
When the supergiant eventually exhausts its core fuel it will explode, hurling its envelope away at speeds of up to 10,000 km/s. The remaining core, or remnant, will be a very dense, compact object. If the remnant is less than 3 solar masses it will be a neutron star (typical mass, 1.4 solar), otherwise it will be a black hole.
Once the expanding supernova shell passes the outermost orbit of the binary, the total mass of the system is reduced. In the majority of cases this mass loss will be less than 50% of the system’s initial total, and therefore the binary may not unbind (but see below). However, the formerly less massive star will now be the more massive of the pair and it will depart its former orbit, leaving on a nearly straight trajectory at close to its orbital speed, thus becoming a runaway. The remnant will be dragged along, but it will occupy a highly eccentric orbit.
Neutron stars can only be observed directly if their beamed radiation sweeps past us as they spin, and as such are called pulsars. Many are found to be racing along at speeds of several hundred km/s. Clearly these are runaway objects, too. Were they formerly single stars or members of a binary, and what could possibly hurl them through space at such high speeds?
The most plausible cause of runaway neutron stars is the so-called “kick” velocity induced by an asymmetry in the supernova explosion. Recall that most neutron stars contain not much more mass than our Sun. Compare this to the 3-20 solar masses of the expelled envelope which was accelerated to thousands of kilometers per second. It’s easy to see how a somewhat off-center supernova explosion could impart a significant velocity to the remnant. Perhaps these asymmetries can arise only in binaries (?), where tidal forces induced by a companion will distort the pre-supernova star.
We’ve now been made aware of the existence of runaway stars, and have some underatanding of their nature. In the next and final installment of this article we’ll meet some easily observed runaways that have interesting stories to tell.
Globular Clusters
The age of globulars can be estimated based on observations combined with physical models of stellar evolution (Chaboyer et al. 1995; 2001). These models incorporate measured and quantum mechanically calculated rates of simple nuclear reactions, basic thermodynamics, elemental abundance estimates based on observations, and the absolute magnitude of RR Lyrae stars (with a magnitude/period relation corrected for metallicity). Using this information, the age of a globular cluster can be accurately estimated based on the main sequence turn off on a Hertzsprung-Russell (H-R) diagram. An H-R diagram plots the absolute magnitude of a star (V) against its colour measured as the difference in magnitude between observations with a blue and a yellow filter (BV). All main sequence stars fall on a curve that slopes from hot young O B stars in the upper left down to red dwarfs in the lower right. As stars age, they run out of hydrogen fuel, and swell up to become red giants, moving to the upper right of the graph. The point of the turn-off determines the age of the cluster. Using this method, the oldest globulars in the Milky Way are known to be 13.2 1.5 GYr old.
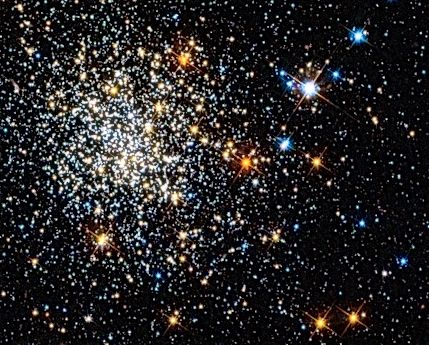
White Dwarfs
White dwarf stars have exhausted their nuclear fuel and glow only from residual heat. The oldest white dwarfs will be the coldest and thus the faintest. By searching for faint white dwarfs, one can estimate the length of time the oldest white dwarfs have been cooling. The age estimate is based on the physics of thermal emission assuming a chemical composition of carbon/oxygen cores, and uniform chemical layering of the white dwarfs due to gravitational sedimentation. This method relies on the assumption that all stars in a globular cluster formed at the same time with an initial mass distribution similar to what is observed.
M4 is 5,600 light-years away in the constellation Scorpius. Hubble’s Wide Field and Planetary Camera 2 made observations of M4 from January through April 2001. This totalled nearly eight days of exposure time over a 67-day period, imaging down to 30th magnitude to detect 75 white dwarfs. Using this method, the age of the cluster is 12.7 0.7 GYr. This measurement is independent from H-R cluster age dating, and puts a similar lower limit on the age of the universe.
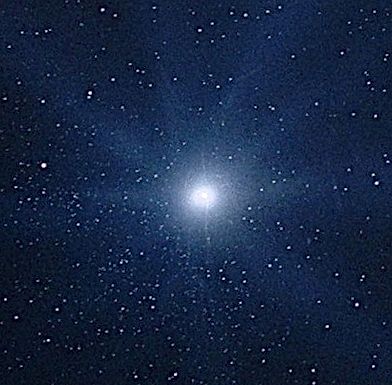