In September and August, respectively, 2002, the Voyager 1 and 2 spacecraft observed their 25th anniversaries in space, were continuing to perform long after their original mission to visit the Jupiter and Saturn systems. After Voyager 1’s encounter with the two gas giants, it was aimed upward out of the plane of the ecliptic. Voyager 2, after its visit at Jupiter and Saturn, was given two more planetary destinations, Uranus and Neptune. It completed its “grand tour” of the outer planets in 1989. It was then aimed downward out of the ecliptic plane. Now, at about 85 AU, Voyager 1 is the most distant human-made object. Round-trip light time is 24 hours. Voyager 2 is at about 68 AU. Their mission now is to study the heliosphere, the vast bubble of space within the Sun’s influence, and the heliopause, the boundary of the solar system with interstellar space. At the heliopause, the outward pressure exerted by the solar wind balances the inward pressure of the interstellar wind. The region where solar wind particles begin piling up against the heliopause is the termination shock, where the solar wind should drop from about 1,500,000 kilometers (nearly 1,000,000 miles) per hour to 400,000 kilometers (250,000 miles) per hour. Voyager 1 is already detecting a slowing of the solar wind from the pressure of inbound interstellar particles leaking through the heliopause. No one knows exactly how much farther Voyager 1 must travel to reach the termination shock or the heliopause.
We Are Grateful to These Canadian Companies Who Have Contributed to Our Efforts
Table of Contents
- We Are Grateful to These Canadian Companies Who Have Contributed to Our Efforts
- Our color and the planets of the Solar System
- Age of the Solar System
- Radioactive Dating of the Universe
- Cosmology
- Biology in Space
- Worlds Beyond Our Solar System
- Doppler Shift Due To Stellar Wobble
- Transit Method
- Microlensing
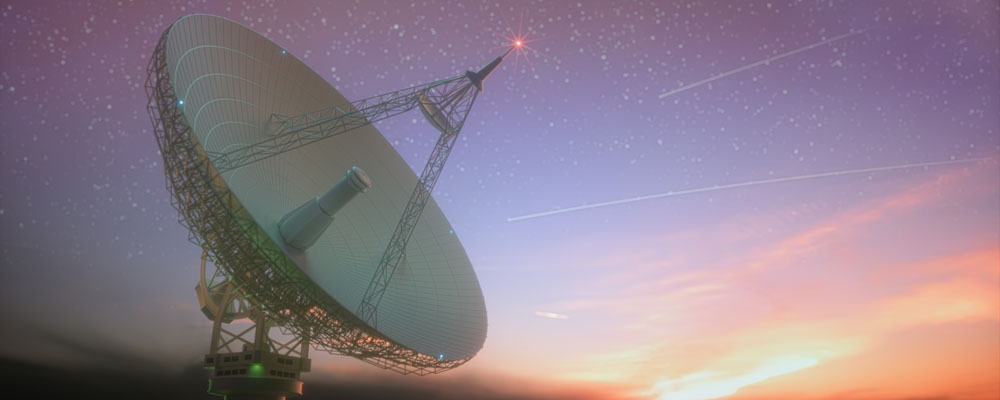
Dr. Ed Stone, Voyager Project Scientist since mission inception, estimates that the spacecraft could reach the termination shock within three years. Once there, Dr. Stone predicts it will still have about 5 billion to 8 billion kilometers (3 billion to5 billion miles) and 10 to 15 years to go before actually crossing the heliopause into interstellar space. Because the heliosphere expands and contracts with the level of solar activity and the inward pressure of the interstellar wind is uncertain, it is very difficult for scientists to estimate the actual extent of the heliosphere.
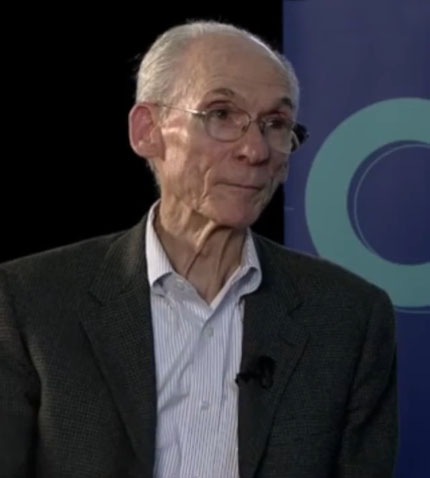
NASA created the Space Place program to give the public the opportunity to explore the space program’s technological advancements and delve into its discoveries. A component of that outreach, the Space Place column is offered to select venues like astronomy associations. In a follow-up message, Nancy explained that she was looking for astronomy clubs that were not just active, but had active outreach programs.
Astro-chemistry, although not as well-known as astrophysics, is a very important field because the chemical composition of the universe is constantly evolving. The Big Bang created only a few light elements such as hydrogen and helium, but further atomic reactions in stars and nebulae have generated the heavy elements. In general, the larger stars contain heavier elements, and studying their spectra provides important clues about the evolution of the universe.
Our color and the planets of the Solar System
You’ve probably heard the old saying that one’s eyesight is the most precious thing to have (as well as your health and well-being). Being able to see gives you the opportunity to stimulate the cones and rods in your retina, a sensory membrane lining the eye’s interior wall. With eyesight, the world around you unfolds; colours are perceived, our sense of depth and perception is stimulated, and everything around you takes on a three-dimensional character.
For the amateur astronomer, vision is a priceless commodity. Especially when it comes to viewing things in the eyepiece. As far as colour is concerned, it is the cones that do all the work. Our brain responds to three primary colours of light; red, green, and blue. These are called ‘additive’ colours. Mixing different combinations of these three colours will give you a smorgasbord of any other colour. For example, if you mix red and green together you’ll get a yellow hue. Put red and blue together and you’ll have purple. There are also ‘subtractive’ colours that relate to paint pigments and dyes. Mixing these will yield a new set of hues. As far as astronomy is concerned, we’ll stick to the former.
The visible light has no colour at all. Colour is created in the mind. Through evolution, our brain has been conditioned to interpret colour the way our brain wants it to be. For animals and other living organisms (including those not of this planet), the concept of colour may be very different. A red car may actually look burgundy or even a shade of blue. It depends on the anatomy and perception of that organism.
Although stars come in a variety of flavours as far as the colour wheel is concerned, no one has come across a green star. At least not yet. The reason why…in a nutshell, there are no stars that radiate wavelengths of light that when mixed produce green. Since green is a primary colour, it cannot be created by mixing. The green hue is found smack in the middle of the visible spectrum. If green would be observed, then both ends of the colour spectrum would have to be cancelled out. In this universe, this just doesn’t happen.
When darkness falls, our colour oriented cones go to sleep and the rods take over. Our perception of colour is greatly diminished and we see only in shades of grey or greenish-grey. When it comes to vision in low lighting conditions, the green wavelength is the colour that the human eye is most sensitive to. That’s why at twilight you can still make out a greenish tinge on the grass. When one peers through a scope to spy a deep-space object, most of the time the predominant hue is a greenish-white. Very rarely would you see shades of blue, magenta, or purple. Of course textbooks and magazines are filled with glitzy images of exotic objects. Only the time exposure gained from an astrophotograph could yield such saturated colours. The problem with newcomers to astronomy is that they read the books first and then expect to see these works of glorious art in the sky. When they have the opportunity to see these same objects through an eyepiece their bubble bursts only to have them packing up their scopes and abandoning the hobby.
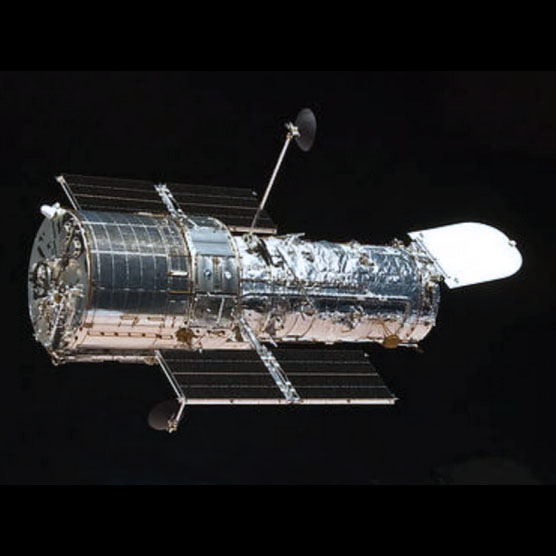
What about the planets we see in our solar system? Except for Mars, Uranus, and Neptune, most planets display bland examples of off-white hues with a hint of colour. If you should desire a saturated pink or peach, consult a Hubble Gallery image from your nearest bookstore or personal computer. No matter how large your backyard scope is, the colour rendition will be about the same. Mind you details will be more prominent in a scope 12 inches or more. If you want some colour in your cosmic carrousel, the planet Mars portrays a vivid creamy orange with a splash of ochre, while the seventh planet, Uranus beholds a mint green. Icy cold Neptune shines with a sapphire blue. Symbolic of it’s remoteness from the sun.
If you’re a meteor lover, you’ll definitely see tinges of yellow, orange, and red and the occasional lime-green bolide. Comet chasers can expect to see similar colours that deep-sky enthusiasts see; greenish-white. The bold blues and the warm yellows are seen in time exposure photographs. Even viewing a comet using high magnification yields a ghostly greenish-white apparition. And finally, as far as auroras are concerned, once again, the greenish hue predominates. This is caused by the ionization of atomic oxygen as the solar wind slams into our atmosphere during a solar storm. When activity becomes high, colours such as magenta, yellow, and purple are seen. But that is rare. Such auroral displays happen only once or twice every 11-year sunspot cycle.
Age of the Solar System
As a limiting data point, we expect that the universe should be older than the objects in it, so an accurate estimate of the age of the solar system can be used as a lower bound. Radioactive dating has shown that the oldest earth rocks are roughly 4.0 billion years (Gyr) old, AstroNotes June July 2007 8 although they contain refractory zircon grains with high melting points that have been dated to 4.3 Gyr. Moon rocks and meteors, however, have been dated to be 4.55 Gyr old. This is not surprising, as most earth rocks have undergone cycles of subduction and melting over the history of the planet, whereas the moon is much less geologically active, preserving much of its original surface geology
The most direct means for calculating the Earth’s age is a Pb/Pb isochron age, derived from samples of the Earth and meteorites. This involves measurement of three isotopes of lead (Pb206, Pb207, and either Pb208 or Pb204). A plot is constructed of the concentration ratios Pb206/Pb204 versus Pb207/Pb204. If the solar system formed from a common pool of matter, which was uniformly distributed in terms of Pb isotope ratios, then the initial plots for all objects from that pool of matter would fall on a single point. Over time, the amounts of Pb206 and Pb207 will change in some samples, as these isotopes are end products of uranium decay (U238 decays to Pb206, and U235 decays to Pb207). This causes the data points to separate from each other. The higher the uranium-to-lead ratio of a rock, the more the Pb206/Pb204 and Pb207/Pb204 values will change with time.
Radioactive Dating of the Universe
One can also estimate the age of the universe by measuring the relative abundance of the radioactive elements that are formed at a known ratio in supernovae. These elements become dispersed in interstellar gas and dust. Radioactive dating methods allow no great precision when applied to a mixed together and evolving system like the gas in the Milky Way. In a gas there is no chemical separation into grains of different crystals, so the absolute values of the isotope ratios have to be used instead of the slopes of a linear isochron fit. This method requires that we estimate how much of each isotope was originally present. This can be done using a physical model for element production combined with the observed supernova frequency.
Trace elements with long half-lives that have been swept up from the interstellar medium and incorporated into the solar system provide the best chronometer. 187Re decays into 187Os with a half-life of 40 Gyr. It looks like 15% of the original 187Re in our solar system has decayed relative to its initial production ratio as given by nucleosynthesis models. This leads to an age estimate of 8-11 Gyr. This, however, is just the mean formation age of the heavy elements in the Solar System, and no rhenium or osmium has been made in the solar system for the last 4.55 Gyr. If all the elements were made in a burst soon after the Big Bang, then the age of the Universe would be 8-11 Gyr by this method. Because the elements are made continuously at a constant rate, the calculated mean age of matter in the Solar System is 11.5-17.5 GYr.
238U and 232Th are both radioactive with half-lives of 4.468 and 14.05 Gyrs respectively. Uranium, however, is underabundant in the Solar System compared to the expected production ratio in supernovae. This is not surprising since the 238U has a shorter half-life. The magnitude of the difference gives another independent estimate for the age of the Universe. Dauphas (2005, Nature, 435, 1203) combines the Solar System 238U:232Th ratio with the ratio observed in very old, metal poor stars to solve simultaneous equations for both the production ratio and the age of the Universe, obtaining 14.5+2.8 -2.2 Gyr
Cosmology
Data from satellites such as the Wilkinson Microwave Anisotropy Probe (WMAP) and its predecessor, the Cosmic Background Explorer (COBE), have provided precise measurements of the fluctuations in the microwave background. This background, the most distant observable photon source in the universe, was first discovered as a uniform radio noise by Penzias and Wilson in 1965. The fluctuations seen by COBE and WMAP represent sound waves echoing through the early universe, imprinted as slight temperature variations in the primordial blackbody spectrum. These miniscule disturbances, currently appearing as ~100 micro-Kelvin ripples on an otherwise nearly perfect 2.728 K surface, are the seeds that eventually coagulated through gravitational collapse into galaxies, galaxy clusters, and supergroups. At the time this light was emitted, 300,000 years after the big bang, the primordial fireball had cooled enough to allow the first atoms to condense, changing the universe from an opaque cloud of plasma to a transparent warm broth of hydrogen and helium. Originally the blackbody spectrum was peaked in the ultraviolet spectral region. Because of the ensuing expansion of the universe, the light has been redshifted through the visible and infrared, all the way down to the microwave spectral region where we observe it today.
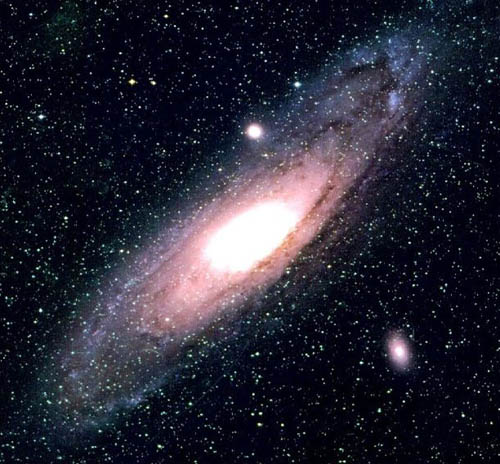
Combined with measurements of the brightness and redshift of distant supernovae (Type Ia), this comprehensive data set strongly constrains the history of the universe. Edwin Hubble first noted that distant galaxies appear to be moving away from us in all directions. Early observations indicated that there was roughly a linear relation between distance and recession velocity. The Hubble constant, H0, was defined to characterize this universal expansion. Until the 1990’s, however, distance estimates of faint galaxies were not sufficiently reliable to accurately calibrate H0. Estimates ranged from 50-100 km/s/MPc, effectively resulting in a factor of 2 uncertainty in the age of the universe. The Hubble space telescope was launched in an effort to solve this problem. Today the Hubble constant is known to be 72 ±7 km/s/MPc, even though 42 would be more aesthetically pleasing to fans of Douglas Adams.
By measuring the statistical properties of the primordial background oscillations, and comparing them to mounds of data on the spatial distribution of galaxies vs. redshift that has been built up over the past several years, cosmologists can now distinguish between the predictions made by various models of the big bang. Cosmological models of universe formation and expansion dominated by normal baryonic matter or hot dark matter are inconsistent with the two data sets. The only well-tested model that fits both the background oscillations and the galaxy distribution data sets results in a 13.7 Gyr old, nearly flat universe that is dominated by cold dark matter, and is undergoing accelerating expansion due to a “cosmological constant”.
In summary, multiple well-supported independent estimates all give consistent results for the age of the universe. We know from at least seven independent radioactive dating systems that minerals in the solar system were formed about 4.6 Gyr ago, consistent with a much older universe. Hubble observations show that the oldest white dwarfs have been cooling for roughly 12.7 Gyr. Models of stellar physics provide evidence that the oldest globular clusters are about 13.2 Gyr old. Our understanding of galactic nucleosynthesis suggests that metals in the solar system are between 11.5 and 17.5 Gyr old. This result has also been derived independently using multiple radioactive decay chains. Cosmological models, constrained by observations of the microwave background and the Hubble expansion arrive at an age of 13.7 Gyr. For the first time ever we have an evidence-based history of creation. Is it possible that we are the first to have gained this appreciation?
Biology in Space
Our galaxy, an average run-of-the-mill conglomeration of about 200 billion stars is only one of about 100 billion galaxies in the observable universe. Using simple mathematics, we can estimate that there are at least 10 billion, trillion stars that our instruments can ‘see’.
Applying current star-formation theories, some of those gazillion stars should have planets around them, Astronomers know that the planet-making process is relatively common, as well as an ongoing event. Planet hunters have located well over 200 worlds within the Sun’s neighbourhood. Many of them gas-giants similar to Jupiter. As planet-seeking techniques become more refined, the objects we find will be smaller. Modern astronomy is on the cusp of finding a twin Earth. Now its only a matter of time.
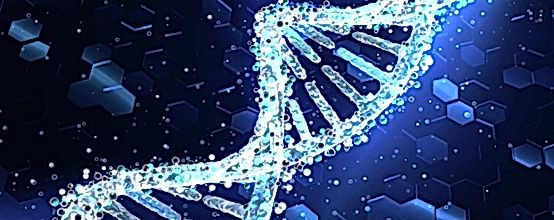
Knowing that carbon-based compounds are commonplace in the galaxy coupled with the fact that the planet-formation process is alive and well, means good news for astro-biologists. What percentage of planets in our galaxy will be capable of accommodating life as we know it? Unfortunately, that is a daunting question. So far, we know of only one planet teeming with life: Earth. Are we the only example of paradise in this corner of the galaxy?
According to Terence Dickinson, the odds are that the evolution of intelligence has occurred more than once. Given the age of our universe, most alien life should have gotten a head start on our civilization. Our only laboratory we can work with is Earth. Assumptions and educated guesses about biology elsewhere are our best bets. Gathering data from the tools we have and using our knowledge about life on this planet should give scientists a head start. Bodies of water and land masses are the basic prerequisites needed for any organism. A candidate planet would most likely orbit a star that mimics our Sun. Although an Earthlike world orbiting a red dwarf is an entertaining thought. These tiny suns are almost eternal giving the process of life ample opportunity to develop, evolve and diversify. Red dwarfs are also known for their gigantic flares. This may pose a problem for any budding life form. But can they adapt such as life on Earth? Given the resiliency of life, it is possible.
Finding life on a planet that orbits a massive star is remote if not impossible. These heavyweights seldom last more than 10 million years. From our limited knowledge we can assume that any organism will not have enough time to develop in such a brief time span before the star blows itself to smithereens. An Earthlike world would find it hard to develop in a system with a Jupiter-class planet close to its star. Smaller bodies would be subject to orbital instabilities inflicted by these behemoths.
Another scenario may play out a role where a terrestrial planet may orbit a Jovian body. In our solar system, classic examples are moons around Jupiter and Saturn. Although conditions on these moons are hostile, to say the least, we can see that the likelihood of smaller bodies orbiting a larger one is possible.
Today there are many theories about the planet formation process, their size, mass, and orbits. Our solar system is the only tangible model we can work with. The question is, is our Sun’s family the norm, or just one model of many out there? We know there are systems bearing sun-like stars. Planetary systems that are vastly different from one another. Some have planets that barely skim their star’s corona, others have planets with highly elliptical orbits, still others have planets that orbit much farther than Neptune does around our Sun. And of course, we’re familiar with those ‘super Jupiters’, worlds that dwarf our own Jupiter.
What are the prospects of having an organism inhabiting just one of those worlds? The idea of life on other worlds has always been a thorny issue. Here at home in our solar system, there is very little hope of bumping into anything more developed than microscopic bacteria. Scientists continue to speculate that primitive life may exist under Mars topsoil as well as in the subsurface salty ocean on Europa.
Given the age of our galaxy, the odds are heavily stacked against two civilizations in the Milky Way that have similar technologies. Chances are they are either way ahead of us or very primitive. Dickinson and the late Carl Sagan state that given time and appropriate technology the feasibility of interstellar travel can be achieved. Let’s use our society as an example. We have carried on space exploration for about 50 years and have successfully sent spacecraft to the outer realms of our solar system. We’ve sent humans to the moon, and within a few decades, if all goes well, people will walk on Mars. In fact, two space probes. Pioneers 1 and 2 have left our system and will soon be in interstellar space. Applying the same analogy, imagine an alien race only 1,000 years ahead of ours and the cosmic magic they can do. Anything more advanced would be staggering to our primitive minds.
On Earth, medical advances are slowly retarding the aging process, we’re using artificial technology to replace parts of our body. Will there come a day when our society can achieve semi-immortality? Living several hundred years would be an asset for long-term space travel. One day will our decedents become part silicon, and part carbon-based? Are we safe to assume that sentient beings from a distant star have already followed this path? Again, a difficult question.
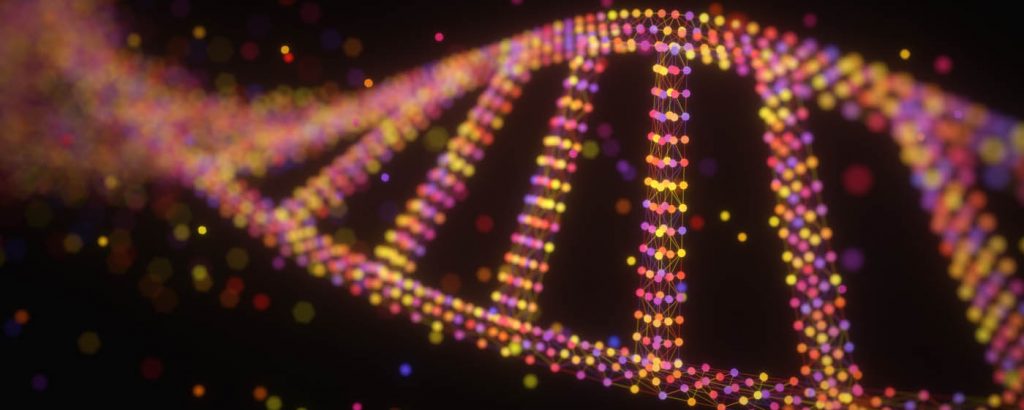
What role will DNA play in other organisms? How will they adapt and evolve in other environments? Will they use their brains much as we have throughout our history? Perhaps other societies are ‘closed’. The thought of exploring space and the great unknown is not as important as looking after the welfare of themselves. Survival comes first, cosmic curiosities come later. It is evident that if intelligent life has taken a foothold elsewhere, given the right circumstances, any advanced race will eventually cross paths with another. If we use our brains in the manner we think and function, then it is logical to surmise that other beings will explore and map their interstellar neighbourhood as well.
Life has taken a foothold on this planet for about 3 billion years. Starting with invertebrates in our oceans then moving onto landmasses. Would this give sufficient time for aliens to notice us?
All issues have their cons as well. Could a civilization destroy itself through nuclear warfare? Is there a self-destruct mechanism inherent in every organism? Perhaps they are hiding in the interstellar medium and have yet to find us. The latter seems a likely bet. Unless an advanced race masters the time-dilation effect by traveling near light-speed, it would be difficult to bump into one another. Unless intelligent life can reside within the confines of a crowded cluster where distances between stars are within a light-year, then a technology would need the perfect spaceship.
The ‘we are alone’ scenario is difficult to imagine. This is a no-brainer. Looking at the majesty of the heavens is enough to convince most of us that life on Earth is not a fluke.
What about a variation of the ‘we are alone’ theme? Life in distant pockets of the universe is always emerging, but never survives as a consequence of natural events. Although resilient and adaptable, alien life, primitive or not, succumbs to a massive meteorite deluge, a nearby supernova, or a collision with a large comet or asteroid. In the past, our planet lay victim to at least five major extinctions. But through it all, I’m here writing this essay. We should thank our lucky stars that a planet as large as Jupiter lies relatively close to us. Some astronomers call it the ‘guardian’ planet. And for good reason. Without Jupiter the solar system’s inner planets would be open to attack by asteroids and comets.
Jupiter’s large gravitational field acts like a magnet keeping foreign objects at bay. If a stellar system is void of such a heavyweight, can a smaller planet be a sitting duck in this cosmic arcade? Can humanity be thankful for the existence of gas-giants?
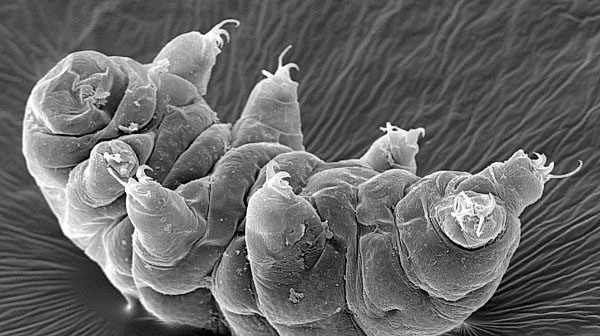
Earth’s only natural satellite, the Moon keeps our seasons in check. Without it the planet’s axis will swing wildly causing extreme climate changes. Will life be capable of adapting to these shifts? The prerequisites for a stable system seem to be endless. Everything must be tweaked just right for the miracle of life to happen. Although fragile, life can be tenacious and it can adapt readily. Extremophiles here on Earth have proved this point. The search for life will continue to become more intriguing as astronomer’s tools become more sensitive and powerful. Perhaps we’ll come face-to-face with something that will knock our socks off. If we as citizens of Earth decide to lay back and do nothing, then we’ll never know for sure.
Worlds Beyond Our Solar System
One of the most exciting areas of astronomy involves the search for worlds beyond our solar system. Eleven years in the making, astronomers have discovered at least 200 planets in 160 stellar systems. Most of these planets are within 200 light-years of Earth. The stories continue to get juicer as planet-hunting techniques become more refined and advanced. We are literally on the cusp of finding worlds similar to our own. One day, perhaps within our lifetime, we will know if the biological process is happening on a distant planet around another sun.
In 400 BC the famous Greek philosopher, Demoritus taught his students of other diverse worlds with life on them. As you can see, this notion has been around for a long time. It is not new.
Upon doing some research on the internet, I discovered that more than 75 programs, ground-based and spaced-based are in the works for finding extra solar planets. The field is blossoming like an apple tree, each apple representing a newfound planet.
The birth of extra solar planet-hunting began in 1995 with the discovery of a Jupiter-mass object orbiting a Sun-like star in the constellation Pegasus. Technically, the first ever planets were found in orbit around a pulsar, of all places, in 1992 by Aleksander Wolszczan and Dale Frail. In fact, further calculations indicate that as many as four objects may orbit the pulsar. The fourth object is perhaps either a comet or a large asteroid.
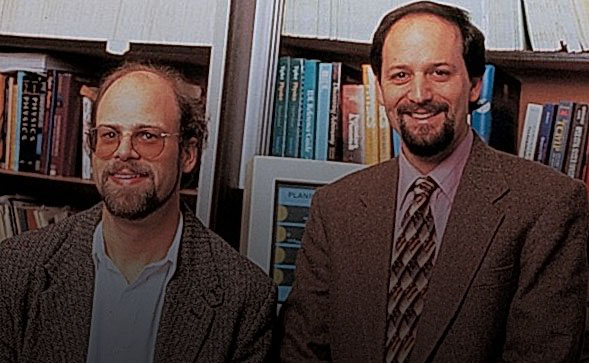
Shortly after Paul Butler and Geoff Marcy (then at San Francisco State University) discovered the Jupiter-mass planet in Pegasus, within three months of their initial discovery, both happened upon a second and third planets around Sun-like stars 47 Ursae Majoris and 70 Virginis respectively. The flood gates had opened with a barrage of discoveries that made their way into the press. A new field of modern astronomy, the detection and classification of extra solar planets, was born.
The face of astronomy would change forever.
As detection methods became more refined, planets with smaller and smaller masses were catalogued. Now the world is waiting for the discovery of another Earth. And in the not-to-distant future, instruments on Earth and in space will be able to tease signatures of biological activity. Not only will we know if life processes occur elsewhere in the Universe, our technology will also permit us to image whole continents on alien worlds. Something that which can only be conceived in science-fiction novels. Planet-imaging still has a long way to go, but for the time being, let us concern ourselves with science that is more tangible and closer to home.
As far as detection methods go, the radial-velocity method seems to be popular. About 90% of known planets were found using this approach. When a planet orbits its host star, it imparts a tiny wobble upon it. This minute wobble can be detected by today’s technology using the star’s spectral signature. Here’s how it works. As the candidate star moves closer to us, its dark lines begin to shift toward the blue end of the spectrum. When it begins to recede from Earth, the lines move toward the red end. These subtle changes allow astronomers to deduce a planet’s minimum mass only. To find a planet’s true mass, it’s orbital inclination must be determined. This method works well for locating large Jupiter-type planets close to their parent stars. One drawback with this approach is that with planets in large orbits, observations must stretch over extended periods.
Doppler Shift Due To Stellar Wobble
The transit method involves the dark disk of a planet crossing the face of its star. Starlight begins to drop very slightly as the candidate object parades slowly across the star’s disk. If dips occur regularly and produce the same dimming each time, then chances are it will be a planet. There is one prerequisite with this method, the star should not be a periodic variable, or change it’s luminosity from causes other than a transiting planet. One advantage of this approach is that a planet’s orbital inclination is known, and combined with the radial-velocity method will give the planet’s real mass. Unfortunately, only a small fraction of stars have planets that cross their disks.
Transit Method
If a star or planet passes in front of a more distant star, the intervening object’s gravity will act as a lens causing the background star to brighten. Today, smaller robotic scopes monitor many distant stars to tease out these microlensing events. Microlensing works with galaxy clusters as well. Matter, both visible and unseen around galaxy clusters distorts space/time causing a more distant galaxy to be gravitationally lensed.
A smooth rise and fall in a distant star’s brightness tells astronomers that a dim star is the intervening star has an orbiting planet around it. With existing tools, Earth-mass planets can be found with this strategy. This approach has a downside, however. Microlensing events result from chance alignments, therefore, they will never repeat.
Microlensing
Direct-imaging is the simplest method of detecting small planets. But it can only be used in infrared wavelengths. Through instruments, starlight is overpowering bright. Any planet would be hopelessly lost in its glare. A popular analogy is the mosquito in the streetlight glare. Try picking out a tiny insect in the glare of a streetlight several miles away. Astronomers are faced with this dilemma.
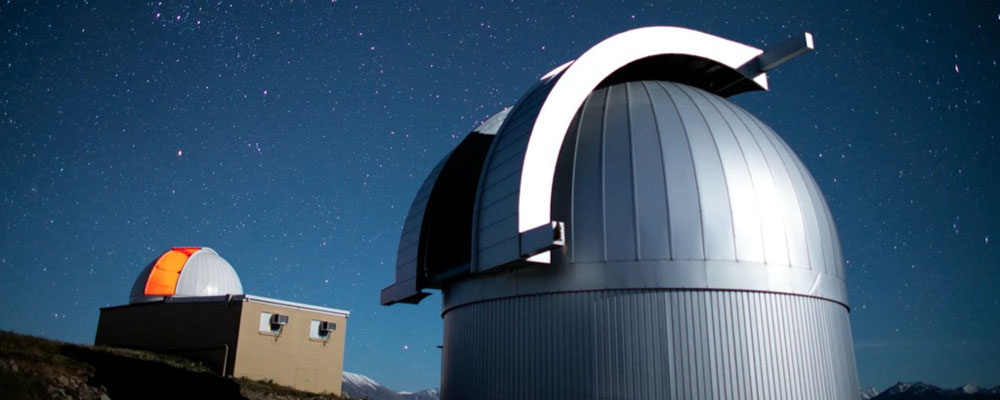
Imaging in the infrared will reduce the star’s glare several millionfold. At the same time, enhancing thermal heat given off the planet in question. Planets emit most of their light in these wavelengths, while stars radiate mostly in the ultraviolet.
About 90 percent of planets discovered thus far travel in elongated orbits about their stars. Gravitational interaction by other planets in the system competing for orbits causes some to get slingshot out of the star’s family, while others may crash into their parent stars.
Closer to home, the planets Jupiter and Saturn may be survivors from a whole bunch of Jupiter-mass planets that pervaded our solar system early in its history. Some stellar systems today may have dozens of planets similar in mass to Jupiter’s. Some will crisscross each others orbits causing chaos and even destruction in the form of planetary collisions. In this scenario it is the survival of the fittest (and fattest).
Scientists think the protoplanetary disk in a young system may also exert a gravitational force upon a fledgling world. Perhaps the nearly circular orbits of planets around their stars may be exception and not the rule. As a system matures, so do the planet’s orbits. A younger system will show signs of disorderly conduct and upheaval while an older one will exhibit order and harmony.
One of the shocking discoveries regarding giant worlds around other stars related to their distances from their suns. Astronomers also noticed many had orbits less than one astronomical unit (AU) from their star. Some of these planet-star distances are only a fraction of the Sun-Earth distance; tight orbits that would defy conventional planet-formation theories. These worlds were appropriately coined “hot Jupiters”. They are both massive and hot. How they formed only several million miles from their star is still largely unknown. One theory states that initially they formed in the cooler, outer reaches of their system and over time migrated inward only to settle there. Another mystery relates to the gaseous atmospheres of these hot Jupiters. Being so close to their stars, why hasn’t their atmospheres burn-up or fizzled away? These are questions planet hunters hope to answer in the future.
To date, most planets found have masses similar to or greater than Jupiter’s. As tools and techniques become more refined, planets with smaller masses (and radii) will start making the list. It is now a question of time when scientists will stumble across a “super Earth.” These objects have masses greater than one Earth but smaller than fourteen Earth masses. In other words, they are larger than Earth, but smaller than Uranus. Their composition remains a mystery.
There are three possibilities to what constitutes a super-Earth. They may be mostly rocky like the four inner planets of our solar system. They could be gaseous and bear resemblances to Jupiter and Saturn. Or they may be both icy and gaseous like Uranus and Neptune. The main aspect that distinguishes a super Earth from another planet is their large and massive rocky core composed of heavy elements like iron, nickel and other silicates. Other planets maybe large and gaseous with a central layer of liquid metallic hydrogen with a small heavy core. These are not considered super-Earths.
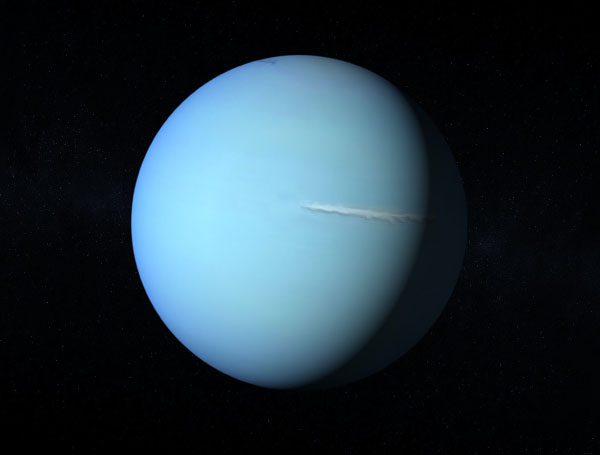
Some known planets may have rocky super-Earth cores. A good candidate is HD 149026b. It has a mass 15 percent greater than Saturn, but a radius is 10 percent smaller. Some unknown mechanism is making the planet heavier for its size. Initial calculations indicate that it has a rocky core between 50 and 70 Earth masses with hydrogen and helium gas making up 30 Earth masses. That is well above the 14 Earth masses required to qualify as a super-Earth.
Astronomers are quite sure that stars rich in heavier elements like calcium, magnesium and iron will likely harbour massive planets. At this point in time, however, formation theories remain weak. Early observations are telling astronomers that it is easier to make smaller worlds than larger ones and that there may be more of them compared to their larger cousins.
Planets with less than 15 Earth masses may form by the accretion process. This theory seems to be a favourite among astronomers. Building planets involves a lengthy multi-step process. Dust particles in the protoplanetary disk begin to clump together with the largest blobs growing faster. The larger sizes allow them to collide more frequently with other dust particles. These dust and ice blobs become planetesimals or “baby” planets. The largest planetesimals gravitationally attract the smaller ones and then the growth rate becomes a runaway process.
Rich planetesimals evolve into Mars-size planets, objects about half the size of Earth. This process alone takes about 10 million years. A young star may have as many as dozens orbiting around it. With the passage of time, these Mars-size objects begin to collide with one another, and crushing upon impact, they stick and grow into objects the size of Earth. It will take anywhere from 20 to 40 million years for this process to take place.
Further impacts can eject magma and create a moon around an Earth-like planet. This is a widely accepted scenario regarding the formation process of Earth’s Moon several billion years ago. Eons ago a Mars-size object grazed our planet ejecting magma and other debris into space. For the next hundred years or so, this debris formed a ring system around Earth. Over time, material in the ring gathered together to form our only satellite.
The bottom line is that stars rich in heavier elements will give birth to large rocky worlds and protoplanetary disks abundant with silicate dust are more likely to form super-Earths.
The “ice-line” in any stellar system is the boundary point dictating whether water will remain in liquid form or turn into ice crystals. Planets with larger than average cores will form with the disk’s ice line about two AUs from their stars. That distance is equal to that of the beginning of our asteroid belt from the Sun. Here temperatures are too high for ice crystals to form. Ice-rich asteroids may deliver water to these worlds. Any Jupiter-like planets will disturb water-bearing bodies causing them to crash into terrestrial worlds. Some planets may receive very little water creating dry desert-like conditions. Others may be literally water worlds covered with huge expanses of oceans
Planets that form outside the ice-line will have the same ice to rock ratios because ice particles will dominate that area of the stellar system. Some smaller examples within our solar system include the moons Callisto, Dione, and Enceladus.
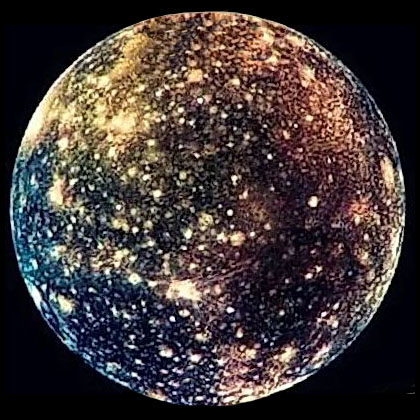
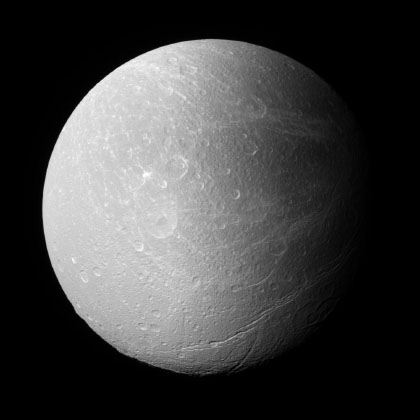
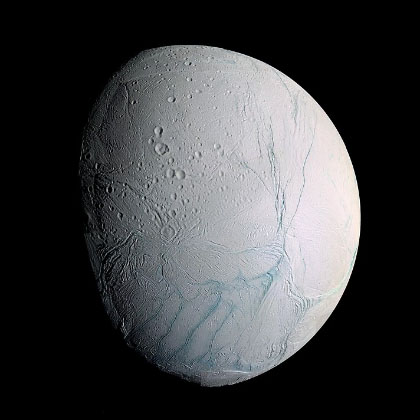
When astronomers begin discovering worlds beyond our Sun’s family, will realize how diverse and alien they will be. The planet-hunting quest has barely begun, and as varied as these worlds may be, one day, perhaps in our lifetime, humanity will come to know just how diverse the process of life can be.