A Traveler’s Guide to Mars
Despite its small size, about half the size of Earth, Mars has some incredibly large geological features. These include the highest mountain, the largest rift valley and the largest impact basin. As amateur astronomers, when we look at Mars through our telescopes, we hope that steady seeing will allow us to make out some of its surface markings. These usually look like dark blotches on a red to orange disk, best seen in the Martian southern hemisphere, or the white polar ice caps. Although pictures taken by Hubble are sharper than the best Earth-bound picture taken by the Lowell Observatory, they can only show dark patches and ice caps. On the other hand, various satellites, from Viking and Mariner to the more recent Orbiter series, have taken detailed photos of a range of amazing topographical features such as volcanoes and valleys. So what’s the link between what we see through our telescopes and the planetary geology of Mars? Well, except for the ice caps, none! This is pretty depressing, so let me explain. Topographic maps of Mars map highlight two principal terrains: the heavily cratered terrain in the South, and the smooth Northern Plains. These are the first order divisions, but let’s move to the next level of detail.
Table of Contents
- A Traveler’s Guide to Mars
- Geological Evidence for the Martian Environment
- Northern Plains
- The Mariner Valley on Mars
- Volcanoes
- Light and Dark on Mars?
- Spirit and Opportunity NASA’s quest for Martian water
The point that the dark markings that we can see through telescopes, from amateur to Hubble, do not correspond to the major topographic features on Mars. The red color is due to fine, oxidized sand, and that the dark markings are areas where that sand has been blown away by the prevailing winds. Perhaps the best-known example of this is the famous dark patch, Syrtis Major, that sits mostly within the Heavily Cratered Highland Terrain, and overlaps onto the smooth plains. In other words, the boundaries of Syrtis Major don’t correspond with any topographic feature: it’s just a large patch of wind-scoured ground!
Scientist Hartmann provides extensive geological evidence and illustrations for the existence of Martian permafrost, or underground ice. In particular, he shows images of a special kind of crater that formed when a meteroid impacted into ice-locked ground and converted the permafrost and its host rock into a muddy slurry that was flung out from the impact crater and draped itself around a pre-existing hill.
However, he also presents evidence for permafrost that I was totally unaware of: Softened Terrain. This is a fascinating concept built around the observation that, in some parts of Mars, what should be high walls and deep floors of relatively large impact craters are way too shallow for their size. They look as though the crater walls have melted and sunk, and as though the crater floors have been filled in by something that is now very smooth. In fact, they look as though they were made of ice cream that had started to melt and started to flow; hence the term Softened Terrain.
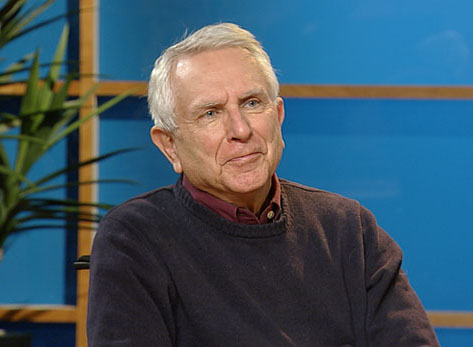
Hartmann’s explanation, supported with excellent photographs, is that these impact craters formed in ground that was underlain by permafrost being affected by a period of Martian global warming. He shows a series of pictures in which impact craters are in various states of definition, from sharply defined to barely visible. It appears that older craters are less well defined, while younger ones are sharper. The interpretation is that the crater walls sank into the ground when the permafrost was warm and soft. In addition, the observation that older craters have sunk more, while younger craters have sunk progressively less, tells us that the impacts that produced the craters must have occurred while the permafrost was soft, during a period of planetary warming. What’s really fascinating is that the best examples of Softened Terrain all come from an area on Mars where cratering statistics indicate that the impacts are older than about 3.5 billion years. This means that this is evidence for a very old period of global warming, which is therefore not just a recent terrestrial phenomenon.
Hartmann presents evidence for what he calls “The Great Waterway,” a giant channel that connects the huge impact basin Argyre and the eastern end of the Mariner Valley where it opens out in a series of braided channels onto the Northern Plains in an area known as Chryse Planitia. The general idea is that water flowed northwards along this Great Waterway during the very early history of Mars, again more than 3.5 4.0 billion years ago, when the Martian atmosphere was dense enough to maintain free water on the planet’s surface.
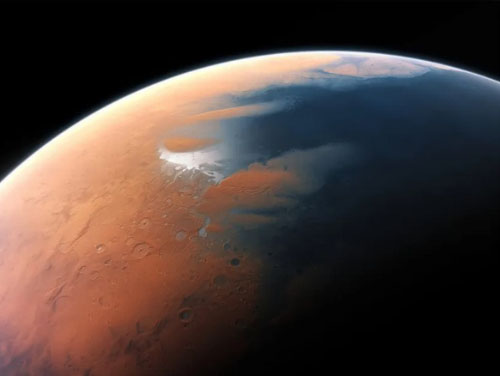
Talking of water, some have suggested that the Northern Plains could have been the floor to an ancient, long-vanished northern Martian ocean. Hartmann presents the pros and cons of the still unresolved debate regarding a possible southern shoreline to that ocean. He even has a photo of a possible sea cliff surrounding a possible bay in the possible coastline.
Mars has lots of valleys, and Hartmann dedicates a significant section of the book to them. He presents an excellent detailed discussion of the evidence for flowing surface water in various river beds that would have emptied into Chryse Planitia at the eastern end of the Mariner Valley. In particular, he presents the valleys Nanedi Vallis and Nirgal Vallis that lie North and South of the east end of the Mariner Valley, respectively.
These valleys are strange because, although they meander like rivers here on Earth, they have no tributaries. Hence the question is, where did the water that carved them come from? This has led some planetary geologists to conclude that, while there may be some evidence for surface water having flowed within these valleys at some stage, the valleys themselves resulted from the release of local groundwater. Surprisingly, despite the fact that there are no equivalents to these valleys on Earth, Hartmann glosses over this and treats the Martian features as normal river valleys.
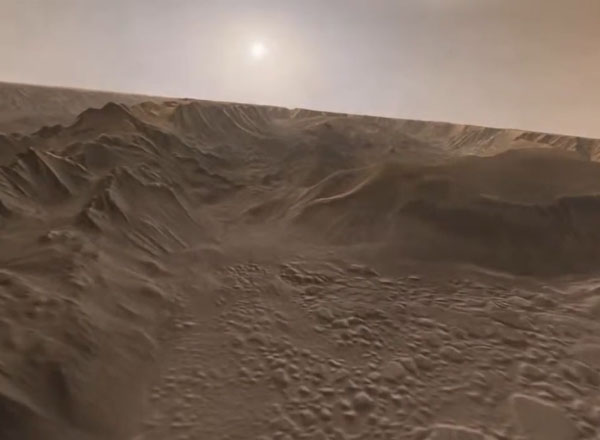
On the subject of Valleys, the Mariner Valley is the longest and largest valley in the entire Solar System. On Earth, it would stretch right across the whole width of the US! Some have described this as a rift valley, but as we know from the Ottawa Valley where we live, in order for a rift to form the valley walls must move away from each other, across the valley trend, in order to allow the valley floor to drop down. That means that the planet has to make room for the valley to widen. On Earth, this is possible because of plate tectonics, where the plates of the Earth’s outer layers can move sideways. But there’s no evidence for plate tectonics on Mars, so there’s quite a debate about exactly how the Mariner Valley formed in the first place.
The Mariner Valley started as a series of fractures whose walls moved parallel to the fractures, not perpendicular to them. This would create long lines of weakness that could be exploited by massive flash floods that carved out the valley by erosion. Hartmann examines this hypothesis in detail and presents evidence for these kinds of fractures elsewhere on Mars.
Tharsis is the strangest geological feature in the entire Solar System; in fact, it defies our understanding of planetary geology. It is as high as the highest plateau on Earth (the Tibetan Plateau north of the Himalayas) and capped by three giant volcanoes, each one twice the height of Everest! The strange thing about Tharsis and its volcanoes is that it’s the only major volcanic centre on Mars, and it has been active in the same place for almost 4 billion years! Compare that with Earth, where volcanism is scattered all over the globe, and only lasts a few tens of millions of years in any one place. But Hartmann really doesn’t do justice to this incredible geological phenomenon. In fact, he doesn’t even mention how incredibly special it is, nor what it means in terms of challenging our understanding of planetary geological theory.
Geological Evidence for the Martian Environment
Planetary geologists are still struggling to understand the huge volcanoes and giant valleys that dominate the Martian topography. But it’s the Martian environment that really fires most people’s imagination. Mention the Martian environment to most people and they immediately think “Water!” especially after the new Mars Odyssey results about permafrost were announced in the general press recently. So, let’s look at the geological evidence for surface water on Mars.
Mars is divided into ancient heavily cratered Highlands in the South, and the Northern Plains that must be relatively young because they have so few impact craters. Some have speculated that the Northern Plains represent planet-scale resurfacing of the northern hemisphere when a huge volume of basalt magma erupted to fill what they interpret to be the largest impact basin in the Solar System, several orders of magnitude larger than the Hellas Basin in the Martian southern hemisphere. Others have speculated that the Northern Plains represent the floor to an ocean basin, just like basaltic ocean floors on Earth. Recently, some planetary geologists got really excited when one of the orbiters1 found magnetic stripes in the southern hemisphere, because magnetic stripes in terrestrial ocean floors provided the first confirmation of plate tectonics on Earth.
The Earth’s magnetic field reverses every couple of millions of years, which means that magnetic north and south simply switch over. When continents move apart during plate tectonics, volcanic rocks crystallise along the mid-ocean ridges that separate the two halves of the ocean bed that are moving apart and creating new ocean floor. These volcanic rocks contain magnetic minerals that record the Earth’s magnetic field at the time of their formation, so rocks that crystallise at different times will have different magnetic polarities. Thus, if we map the floor of the Atlantic Ocean for example, we find that it has alternating magnetic stripes, aligned N-S, parallel to the mid-ocean ridge.
On Mars, the orbiter found something similar, and some people jumped to the conclusion that magnetic stripes mean plate tectonics, ocean floors, and therefore ocean water! However, if you take a good look at the Martian magnetic stripes, they are not symmetrical, whereas they should be according to the plate tectonics model.
Therefore, there is not see any evidence for a Martian mid-ocean ridge from which the two halves of an ocean floor would have grown as the planetary magnetic field reversed every once in a while. 1 This discovery was made with Mars Global Surveyor when the spacecraft was in its first modified aerobraking phase.
Northern Plains
Streamlines made by flash floods carrying sand that flowed around craters in the Northern Plains are taken by planetary geologists as the primary evidence for the presence of water on Mars.
The small size of the few impact craters that affect the flash flood deposits indicates that the Northern Plains are relatively young.
However, there’s much better geological evidence for surface water on Mars, without speculating about planetary oceans. For example, there’s the now famous image of the streamlining created by some fluid, most likely water, that flowed from the Highlands onto Northern Plains, sculpting the sediments (probably sand) that accumulated around impact craters. The impact craters were already there when the sand was deposited, but the sand itself shows no craters, which tells us that the sand and the water that deposited it were relatively young (<3.8 billion years!)
The big debate these days is just how young these features, potentially created by running surface water, really are. Are they billions of years old, or could they represent geologically very young features, perhaps as young as 10 million years old? There’s not much doubt that some channel systems are ancient. Some impact craters appear to truncate the upper reaches of channels, suggesting that the channels are older than ~3.8 billion years, because the great meteorite bombardment is thought to have ended about then.
What about surface water on Mars in geologically recent time, say the last 10 or 100 million years? This idea presents some enormous problems because of the very thin Martian atmosphere. At low atmospheric pressures, water turns into water vapour very easily, even at low temperatures. In a Martian atmosphere, 100’s of times thinner than the Earth’s, the scientific estimates are that surface water cannot survive for more than a few minutes! This means is that any geologically recent features created by surface water on Mars must have involved really fast flash floods. It also means that if standing water, such as a sea or an ocean, was able to survive on Mars for any extended periods of time, the atmosphere of Mars must’ve been much thicker than it is today in the early history of the planet. What are the visible signs that tell us that Mars has an atmosphere? First, we can see clouds that form over the tops of the highest volcanoes, just like clouds that form over high ground on Earth.
Second, we can see “sand” dunes in high resolution pictures taken by the various Mars Orbiters. Dunes form and migrate when wind (which is evidence for an atmosphere) blows sand up the gently dipping slope and over the crest. The sand grains accumulate in the lee of the wind on the steep slopes. Knowing this, we can tell which way the Martian wind blows. Furthermore, some dunes appear to be very young, as we can tell by observing that they cover some impact craters, but show no sign of impact cratering themselves.
So, Mars appears to be a dry, dusty place where water can’t last long at the surface. Yet we keep hearing about water on Mars; so where is it hiding? Well, Mars is also pretty cold with an average daytime high of about _50°C, leading most planetary geologists to think that Martian water is locked up in the ground as permafrost. Characteristic Martian splosh craters that look like mud splashes constitute primary geological evidence for permafrost. Some planetary geologists think that Martian polygonal ground patterns are also characteristic of permafrost. While there’s no doubt that similar polygonal shapes are found in permafrost terrains on Earth, the Martian ones are up to 3 orders of magnitude larger than their terrestrial equivalents, so their interpretation remains ambiguous.
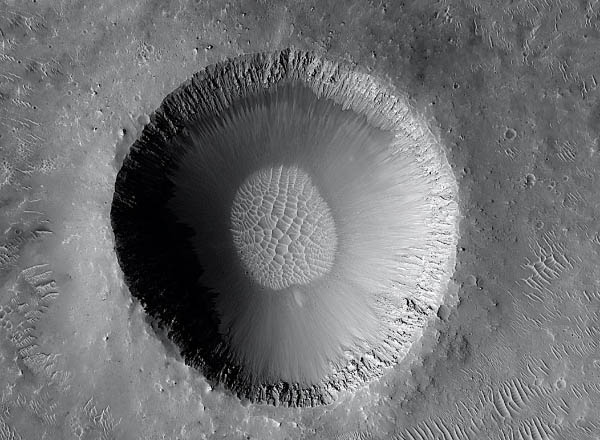
Whatever, it was no surprise to planetary geologists when the media highlighted the recent “discovery” of “water” around the south pole by the Odyssey satellite, now in orbit around Mars. In reality, what Odyssey detected was evidence for hydrogen. This is most reasonably interpreted as the result of water ice in the form of permafrost very close to the surface. However, the real significance of the Odyssey data is that they confirmed the existence of permafrost on Mars, and showed us where it is presently concentrated. Which brings us to the polar ice caps.
The Martian polar ice caps are very different from their equivalents on Earth. Basically, the northern ice cap is mostly made of water ice, while the southern one contains a lot of frozen CO2. The northern ice cap is large and thick, up to a kilometre or so thick with a bit of CO2 frosting, while the southern ice cap is smaller, thinner, and shows large variations in size from season to season due to the formation and sublimation of a widespread, but very thin layer of frozen CO2, only 10’s of centimetres thick. This is one of the most fascinating features of Mars that was explained in a short article in the April issue of the RASC Journal as due to two main factors: summers are hotter and winters are colder in the southern hemisphere as compared to the northern hemisphere, and Martian topography is higher in the south than in the north.
As on Earth, seasons occur on Mars because the planet’s rotation axis is tilted with respect to the ecliptic, which is the plane in which the planets rotate around the Sun. Furthermore, the Martian orbit around the Sun is slightly elliptical, such that summer in the southern hemisphere coincides with the time when Mars is at its closest point to the Sun. Therefore, summers are hottest and winters are coldest in the southern hemisphere on Mars. What this means for the Martian southern polar ice cap is that lots of water ice turns into water vapour in the summer, and is replaced by frozen carbon dioxide frost in the winter.
So, the question now is; “where does that lost water ice go?” Answer: it goes down hill! Remember that the Martian southern hemisphere is dominated by Heavily Cratered Highlands, while the northern hemisphere contains the Northern Plains or lowlands.
According to new planetary climate modeling, this difference in topography drives planetary air circulation from the south toward the north, carrying the water vapour away from the southern polar ice cap and depositing it as water ice at the north pole. The big question here is how long has this weather system been the way we see it today? Is this the explanation for the concentration of water ice in the northern polar ice cap?
Channels leading into the Northern Plains (top right region) look like river systems with tributaries converging downstream. The impact craters appear to truncate some channels. But there are no crates in the plain!
The Mariner Valley on Mars
The Mariner Valley on Mars is not the longest and largest valley in the Solar System, and points to the Mediterranean Basin and the Atlantic Ocean Basin as contenders for this title. In so doing he raises an interesting point: what do geologists mean when they speak of valleys? The term refers to elongate topographic depressions. In the case of glacial valleys, river valleys and wadis, they are formed by the erosional effect of flowing ice, running water, or flash floods, respectively. Rift valley floors are down-dropped blocks of the same material that forms the high-standing valley walls, commonly covered by a thin veneer of younger sediments or rocks. While the Mariner Valley is comparable in length with the Great Rift Valley of Africa, the latter is significantly narrower with an average width of 100 km, compared with 250 km for the former. At its widest, the Mariner Valley is 400 km across at Melas Chasma, even discounting the contiguous Condor Chasma branch on its north side. It is also 400 km wide at its eastern end (Eos Chasma). Geometry aside, most planetary geologists consider Mariner Valley to be an erosional feature, rather than a rift.
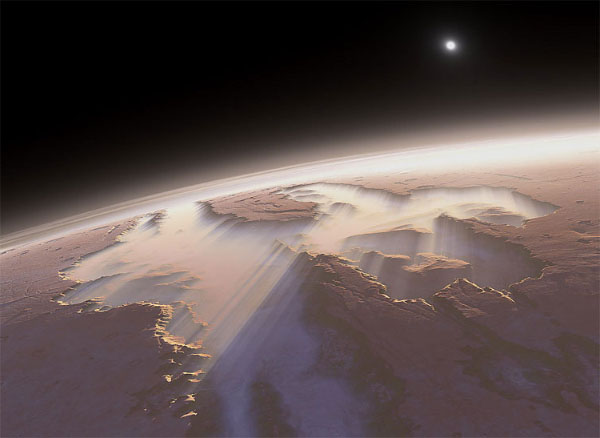
Ocean floor and continents on Earth with the water and vegetation removed. Note the segmented mid-ocean ridge in the middle of the Atlantic Ocean. Similar segmentation is even better developed in the eastern Pacific Ocean, especially to the south. Note also the jumble of oceanic crust and continental material in the Mediterranean Basin between Africa and Europe.
Now consider the Atlantic Ocean Basin. It is indeed flanked by the American, European and African continents. However, it is not an erosional feature, and its floor is not underlain by continental (Si-Al rich granitic) material. Instead, it is an ocean basin floored by new (Fe-Mg rich basaltic) crust that formed as the flanking continents drifted apart in response to sea-floor spreading. Hence, it is not a valley by any geological definition. Nonetheless, there is a narrow rift valley that runs along the entire length of the mid-ocean ridge that is the site where new oceanic crust is still forming today. However, the width of this rift is two orders of magnitude narrower than the Mariner Valley. More importantly, it is made of many of independent segments whose individual length is measured in hundreds of kilometres, as compared with the 3600+ km length of the Mariner Valley, even discounting the Noctis Labyrinthus sector at its western end.
The Mediterranean Basin is not a valley either. It comprises an extremely complex jumble of oceanic crust and continental nuggets that formed in response to jostling of the European, African and Asian continents that flank the basin. The various “seas” and island chains identified within the Mediterranean correspond geographically to openings in the basin floor into which new oceanic crust rose, but which were arrested in their development before they could become full-fledged ocean basins. This has occurred repeatedly throughout relatively recent geological time, ever since the African and European continents collided to form the Alps and related mountains.
The interesting point raised by Mr Wray’s comment is this: when is a topographic depression not a valley? To which one answer is, when it’s an ocean basin, even a complex failed one. The key to understanding the distinction is the difference in the mechanisms and processes involved in the formation of the different structures: erosion and/or rifting vs continental drift and the formation of new ocean crust through sea-floor spreading. In short, the Mariner Valley is the longest and largest valley in the entire Solar System, until such time as a large, shrouded planetoid such as Titan reveals something even larger.
Volcanoes
Let’s start with the volcanoes associated with the Tharsis Bulge, including Olympus, the largest volcano or mountain anywhere in the Solar System (25_27 km high, compared with less than 10 km for Everest). The Tharsis Bulge is a large plateau highland. Because of the distribution of impact craters that affect the lavas that erupted from the volcanoes, we know that the Tharsis Bulge has been the site of active volcanism for a very long time. The oldest volcanic eruptions, peppered with impact craters, are up to 3.8 billion years old, while the very youngest show no impacts at all, and may be as young as 25 million years old. Think about this for a minute— we’re talking here about a huge highland region, with some of the largest volcanoes known in the Solar System, that have continued to erupt for billions of years, not just in the same place, but in one location on the entire planet! Just how do you do that?
One thing we can be sure of is that there’s nothing like it on Earth! Volcanoes here are scattered all over the planet, and erupt for a few million years at the very most. This is because they are generated by plate tectonics in dynamic environments that are constantly changing. In contrast, the Tharsis volcanoes require a situation that has remained more or less constant for billions of years.
Some planetary geologists suggest that the location of the Tharsis volcanoes, including Olympus, indicates that Martian volcanism is not controlled by plate tectonics as on Earth, but by huge plumes of hot material that rise inside the planet from its core. This could also explain the great height of the Tharsis volcanoes, because they would be sitting on a bulge held up by the same rising plume that brought the hot lava to the surface in the first place. At first blush, this sounds reasonable, until we start asking questions: Why is there only one major volcanic centre? Does this mean there’s only one plume in the entire history of Mars? How does that plume of hot material keep on rising for billions of years? Earth has plumes too, but there are lots of them at any given time in geological history; they die off with time and are replaced by others in different places. Maybe the single plume model isn’t such a good explanation after all. So, what are the alternatives?
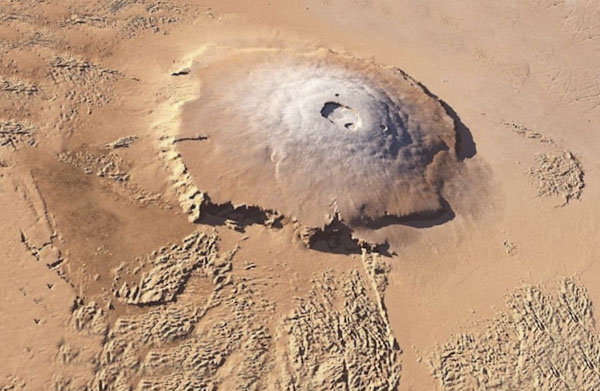
A plume is a column of hot material rising from the planet’s hot core, through the cooler mantle, and up to the cold crust. Other planetary geologists wonder if the interior of Mars had lots of smaller plumes scattered all over the place just like the Earth, and have suggested that the Tharsis bulge is the one place on Mars where the crust is thinner than anywhere else on the planet. They explain the billions of years of volcanism as the result of a local defect in an otherwise normal planet. This sounds OK, until we ask why the volcanoes associated with the Tharsis bulge are so high? For some the answer is pretty simple. The Tharsis bulge with its gigantic volcanoes represents a huge load sitting on the planetary surface without sinking; therefore the Martian crust must be extremely strong in order to suppport it. Geologically, that means that the crust must be extremely thick in order to be so strong, but this is just the opposite of the “thin-crust” hypothesis that interprets Tharsis and Olympus as a leak through a local patch of thin Martian crust.
Tharsis is a huge plateau or bulge that rises gently from both the cratered terrain and the Northern Plains and is peppered with several giant volcanoes. Principal Martian Topographic Features To the West of the Tharsis Bulge is the smaller Elysium bulge which also has volcanoes on it. East of the Tharsis Bulge is a huge valley system called the Valles Marineris in honor of the Mariner probe; we can call it the Mariner Valley. The upper reaches of the valley system start in the SE corner of the Tharsis Bulge, and end at the boundary between the cratered terrain and the Northern Plain. In the southern part of the cratered terrain are two huge elliptical areas with flat interiors called Hellas and Argyre. The volcanoes and valleys are easy enough to understand, but what are the Northern Plains, and what are Hellas and Argyre plains doing in the middle of the cratered terrain? For that matter, what is the cratered terrain anyway? Heavy cratering means “old,” because if those are impact craters then we know that the main period of impact cratering in the Solar System ended before about 3 billion years ago. So, if the Northern Plains show less evidence for impact cratering, then they must be younger than the cratered terrain, just as the smooth lunar mare are younger than the cratered lunar highlands. Therefore, if we extend this line of reasoning, then the Hellas and Argyre Plains are probably impact basins filled with lava.
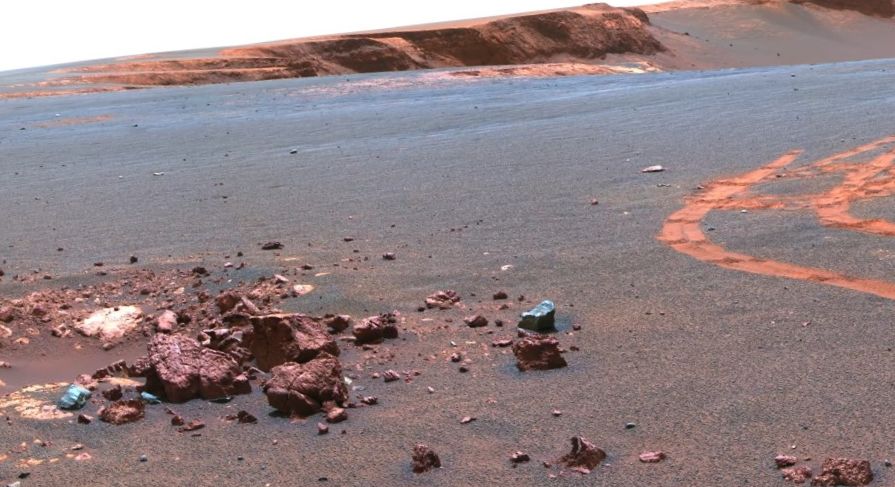
The Tharsis Bulge is topped by a line of three giant volcanoes. Each one has a diameter equivalent to the distance from Ottawa to Montréal! Most importantly, these three volcanoes, and much of the Tharsis Bulge, have no impact craters. This means that the bulge and its volcanoes have been re-surfaced by lava flows after the time of major meteorite bombardment in the Solar System. Some planetary geologists have proposed that the volcanic activity on the Tharsis bulge is extremely young, maybe as young as 25 million years. Olympus is as big as the entire Hawaiian Islands volcanic chain in the Pacific On the SE edge of the Tharsis Bulge, the upper part of the Mariner Valley shows no impact craters, so it must be younger than 3 billion years old too.
How big are the main elements of Martian topography? The biggest volcano on Mars is Olympus Mons, perched on the NW side of the Tharsis Bulge. In terms of its diameter, it’s as big as Hawaii and all of its volcanic neighbors! In other words, it would span from Ottawa to Boston! But Olympus Mons is not just broad in the beam, it’s also incredibly high nearly 3 times higher than Mount Everest, whose peak elevation is a bit less than 10 km, making Olympus Mons about 27 km high (in my talk, the graph I showed incorrectly represented Olympus Mons as only 17 km high). That makes Olympus Mons the largest volcano and the highest mountain in the Solar System! The Mariner Valley would span the entire width of the US. That’s about equivalent to the Great Rift Valley of eastern Africa, but the intensity of its development, as expressed by its branches, and its depth, have no equal in the Solar System. Hellas is the largest impact basin or mare in the Solar System. It is so large, more than 2000 km, it would fill the western and central US! Compare that with about 1200 km for the larger lunar impact basins. Light sand covers dark rocks Nothing here but dark rocks
What we’ve just examined is the greatest puzzle faced by planetary geologists studying Mars. None of these explanations seem to work, and some of them contradict others. The bottom line is that these huge, high, extremely long-lived volcanoes that occur in one spot on an entire planet are one of the great enigmas of the Solar System. We simply don’t know how to explain them yet!
Light and Dark on Mars?
Dark patches we see through telescopes are areas devoid of sand where dark rocks are exposed. The point I’m trying to make is simple. Mars might be a bit on the small size as planets go, but it’s got some pretty impressive topography. So, if Mars is so well endowed physically, how come we don’t see any of this through telescopes not even Hubble? If we’re not seeing this impressive topography through our telescopes, what exactly are we seeing? The dark patch that is most readily observed through amateur telescopes is Syrtis Major, simply because it’s the biggest and darkest patch. However, it is not located near any of the major topographic features that Mars is famous for. Syrtis Major is simply a slightly elevated area in the cratered terrain, near the margin of the Northern Plains. So, why is it so obvious through our telescopes, while the really important features fade into obscurity? It turns out that the colors of the Martian surface are simply related to the distribution of light colored sand versus dark colored volcanic rocks. In other words, what we see through our telescopes is simply a reflection of the distribution of sand on the Martian surface. Syrtis Major is an area where sand has been removed, presumably by wind, and the uniform color of sand over much of the rest of the Martian surface hides the incredible topographic highs and lows that our probes have photographed from Mars orbit.
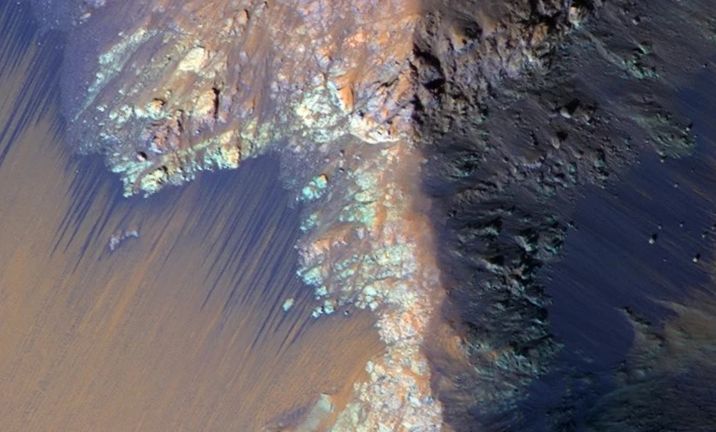
Spirit and Opportunity NASA’s quest for Martian water
There’s no doubt whatsoever that these two Rovers have been an unqualified technical and mechanical success. However, they are supposed to be on a scientific mission investigating the ground-based geological evidence for large, long-lived bodies of standing water seas and oceans in Mars’ early history. Mars may have had seas and ocean’s once upon a time, the best evidence for which comes from images taken by orbiting satellites. However, I suggest that the evidence from the Rovers is equivocal at best, and that in its quest to find evidence for former seas and oceans on Mars, NASA is stepping beyond the bounds of scientific integrity. In short, NASA is pushing interpretations beyond what the geological evidence will allow. Given the extraordinary resemblance between much of the Martian surface today and modern Earth deserts, NASA needs to maintain a high degree of scientific integrity in pursuing its avowed mantra: “Follow the Water”.
Spirit was sent to Gusev crater because the smooth crater floor and the valley breaching the crater wall suggested that it may once have contained a lake of standing water. However, the very first images posted on the web after touchdown clearly showed that something was wrong with this interpretation. Water tends to round large rocks into pebbles as the rocks bang up against each other and get eroded, but there wasn’t a rounded rock in sight. Instead, the images showed widely spaced angular rocks that are either impact ejecta or ventifacts, i.e. rocks faceted by the sand blasting action of desert winds. After a few days, NASA admitted that the lake bottom hypothesis was weak, but then claimed that the fine grained sand between the rocks had blown in from a dried up lake bottom somewhere else!
Shortly after, Opportunity landed in a wide open plain on the other side of the planet called Meridiani Planum, and immediately NASA claimed it had found evidence for the deposition of sediments on an ancient sea floor: “Blueberries”. These are small ball-bearing-like objects made of iron oxide. NASA immediately identified the blueberries as analogous to ooliths that make up limestones that precipitate like pearls from seawater directly onto shallow sea floors here on Earth. The problem with this is that ooliths in limestone are packed together like sardines, not widely separated as are the Martian blueberries. If the blueberries formed by precipitation on a sea floor, then the sediment that contains them now must’ve buried them after they formed. However, commonly the layering in the sediment does not deflect around the blueberries. Instead, the blueberries overgrew the layering and, therefore, must have formed later than the sediment they are contained in. In other words, the blueberries grew inside wet unconsolidated sandy sediment beneath the surface; a very common phenomenon well known on Earth. They indicate the presence of groundwater, but do not constitute evidence for a sea or ocean of standing water at the Martian surface.
Sedimentary rocks contain layers that reflect the processes of sedimentation by which they formed. The Rovers have imaged classical geological features wherein older sedimentary layers are truncated at a clear angle by younger layers that lie on top. In larger-scale versions of this feature, the older layers represent a cross-section eroded through the steep face of a migrating sand-dune-like feature, while the younger layers represent a cross-section of the gentle back slope of another dune that climbed over the first one (hence one can tell “up” and “down”). This kind of layering can form due to the action of wind, volcanic explosions or water which NASA acknowledges, but systematically places the emphasis on the water hypothesis.
On Earth, the classical sedimentary evidence for deposition of sediments on a shallow sea floor is the presence of ripples in the sediment that indicate two diametrically opposed directions of current. Ripples are like miniature sand dunes, so a cross-section through two back-to-back ripples would look like a “smiley mouth” with dimples. In fact, NASA has called what they interpret to be back-to-back ripples on Mars “Smiles”. Unfortunately, the images of symmetrical ripples are not very clear, or very convincing.
NASA has also determined that fractures in rocks can constitute evidence for the presence of standing water on the surface of Mars. NASA interprets what it calls “Razorbacks” as cracks though which water once flowed. This is very reasonable, but the razorback is simply a vein of harder material (quartz?) deposited in the crack, that has subsequently resisted erosion. However, the water that deposited the vein mineralisation was flowing at depths of 100’s to 1000’s of metres below the planetary surface, and it tells us nothing about the presence or absence of standing surface water.
Although this isn’t strictly related to the observations by the Rovers themselves, we can stand NASA’s quest for seas and oceans on Mars on its geological head. Seawater on the surface of a planet warm enough for water to be liquid will try to evaporate, and in doing so it will precipitate calcium carbonate (CaCO3) directly onto the sea floor as thick layers of limestone.
NASA has made a big deal about finding traces of calcium carbonate between the grains of other materials, but underplays the fact that they simply haven’t found any limestone anywhere, using Rovers or orbiting probes. Pursuing this line, the commonest rock on Mars is volcanic basalt that resembles the stuff that Earth’s ocean floors are made of. One of its common minerals is olivine, a mineral well known on Earth. In the presence of abundant water, like a sea or ocean, olivine readily breaks down to something completely different. However, fresh unaltered olivine is very common and very widespread on Mars, as orbiting probes have shown, suggesting widespread, long-term, dry conditions on the Martian surface. NASA acknowledges this, but underplays its significance with respect to the quest for water. Lastly, evaporating seawater in very shallow seas gives rise to two kinds of special chemical sediments: sulphates (e.g. jarosite that NASA has made a big fuss about), but mostly chlorides such as common salt, which is only present as traces on Mars. Once again, NASA underplays the significance of this lack of chlorides in its Martian Sea or ocean model.