Moon. How was it?
The light rock is called anorthosite, principally made of a low density mineral called feldspar. Because the outer part of the Moon, called the crust, is about 70 km thick and is mostly made of feldspar, that’s a lot of lightweight material. Why is the Moon’s crust so light? There’s only one way to do this geologically. At the beginning of its history, the outer part of the Moon must have been completely molten, an ocean of molten rock several hundred kilometers deep! Crystals began to form in this magma ocean as it cooled down and, because crystals of feldspar are so light, they rose like a scum to the top of the ocean, while the heavier crystals like olivine and pyroxene sank toward the bottom. The heat needed to melt the outer part of the Moon probably came from energy released by the impacts of the material that collected together to make the Moon in the first place, about 4.5 billion years ago.
Table of Contents
- Moon. How was it?
- How did the Moon form?
- Visual Evidence for the Evolution of the Moon’s Surface
- Craters Meton and Pitatus
- Total Lunar Eclipse
- Lunar eclipse geometry
- Asymmetrical Moon
- Magma Buoyancy
How did the Moon form?
According to current theory, the chemical compositions of the Earth and Moon can be best explained if the Earth was struck by another planetary body about the size of Mars, very shortly after Earth had first formed. The Moon seems to have very little iron in its core, so planetologists think that the outer part of the impactor, and some of the outer part of the Earth, were literally volatilized and ejected into orbit. There, the ejected material condensed as a ring system around the Earth, somewhat like Saturn’s rings, except that within a few tens of years, the unstable ring system coalesced to form the spherical body that became our Moon.
After the Moon had formed, and the light material had floated to the top of the magma ocean to make the crust, the Moon continued to be bombarded by planetesimals that left many of the scars we see on the lunar surface today. These scars are of two kinds: impact craters and impact basins. Here, the focus is on impact basins because they are the key to understanding the difference between the Highlands and the lunar Mare. What are impact basins? They are so big that planetary geologists have argued for quite a time about whether or not they could be the results of impacts, or whether they were the result of the internal geology of the Moon. Their dimensions can be impressive, up to 1200 kilometers across and 12 kilometers deep. (In comparison, Everest, the highest peak on Earth, is about kilometers high.)
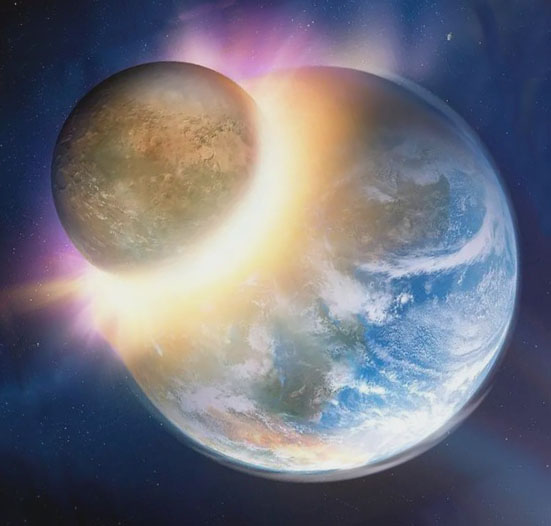
It turns out that they definitely formed from major impacts. Look at the Orientale Basin (not visible from Earth). It has a bull’s-eye shape with concentric ridges around its center. These ridges are circular mountains that Impact Basins, Highlands, and Selected Craters formed when the force of the impact sent out shock waves that lifted and tilted the anorthosite crust at the edges of the huge hole that formed when material from the center of the basin was hurled out by the impact.
Compare this with the Mare Imbrium (the Sea of Rain— easily visible with any Earth-bound telescope), ignoring the dark material for the moment. The Carpathian, Apennine, Caucasus, Alps and Jura mountains form a nearly continuous arc of highlands that are interpreted as the outer-most ridge that resulted from the impact that created the Imbrium Basin in the first place. Lunar planetologists also believe that the LaHire and Spitzbergen Mountains inside the Mare Imbrium form the remnants of an inner ring of impact-related ridges.
The dark material that fills the Mare is made of basalt lava, very similar to the rocks that form the seabed of Earth’s oceans. It’s dark because it contains a lot of the dark mineral pyroxene that is composed of iron and magnesium, as well as silica. The huge impact basins like the Mare Imbrium were flooded by giant basalt eruptions that filled them, but not quite to the brim. Hence we can still see the highland crests poking through.
If you look at a lunar map of the rest of the near side, you’ll see that the Mare Serenitatis (Sea of Serenity) and the Mare Crisium (Sea of Crises) are very similar to the Mare Imbrium, but what about the other large patches of dark material? The Mare Humorum, Mare Nectaris and Mare Fecundatis (Seas of Tears, Nectar and Fertility) are somewhat similar to Imbrium, but have a more corroded look because they are older and have probably been flooded with more lava. However, the Mare Nubium (Sea of Clouds) and the Sea of Tranquillity are so old and have been flooded with so much lava that it is difficult see any trace of their original circular Highland rims.
When were these huge impact basins flooded with basalt lava? The impacts that formed the basins occurred about 4 billion years ago, but the main basalt lavas didn’t erupt until about 3 billion years ago! Why did the lavas erupt so long after the initial impacts? Like Venus, the Moon was always dry. If you check the September AstroNotes, you’ll see that this means that the lunar interior was very stiff, even when it was young and still warm, so plate tectonics could not operate and enable the Moon to cool down from the heat that was gradually building up due to the radioactive breakdown of elements such as uranium and potassium in its interior. Heat built up inside the Moon until melting began at roughly 200-400 kilometers below the surface, and basalt magmas rose to the surface taking the heat with them. However, because the Moon is relatively small, it didn’t contain very much radioactive material, so it couldn’t heat up enough to produce more huge volumes of lava after the Mare had formed.
Next time the Moon is up, and you cannot see your favorite deep sky objects, take another look at the planetary geology of our nearest neighbor. You’ll be looking at the relics of l a Moon-wide magma ocean and the scum that floated to its surface; giant impact structures that formed lunar basins; and the build-up of radioactive heat inside the Moon that led to melting and huge eruptions of lava that flooded the impact basins and almost buried the highlands.
Visual Evidence for the Evolution of the Moon’s Surface
Last month, I pointed out the two principal features we can see on the visible side of our nearest neighbor in the Solar System, apart from the heavily cratered terrain: light colored highlands and dark colored lunar Mare. Let’s change scales of observation and look at some of the surface features that allow us, as amateur astronomers, to determine the kinds of processes that occurred in the Moon’s past, and some of the visual evidence for separating older events from younger ones.
Valleys Lunar craters can be observed with just about any telescope or with steadily-held binoculars, but with a steady telescope, you can see valleys. Lunar valleys come in two varieties: straight and squiggly. The straight ones are usually interpreted as simple rifts whose walls were pulled away from each other so that the valley floor dropped into the resulting space below.
Sirsalis Rille is a nice example of a rift about 450 kilometers long, but it’s not very easy to see from Earth. Other straight valleys are much easier for amateur telescopes, but they’re not quite as simple as suggested by the rift model. For example, the Hyginus and Ariadaeus Rilles are made up of bent or offset segments. However, the really interesting valleys are the squiggly ones, like the example in the Alps on the north side of the crater Plato. Near the crater, it is a continuous squiggly valley, but it seems to feed into a chain of holes and short “squiggly valley” segments. These features are interpreted as ancient lava rivers. Very hot, runny lava was able to flow over the lunar surface for long distances before it froze. As it cooled, it solidified on the outside first, forming a long, skinny tunnel that still-molten lava could continue to flow through.
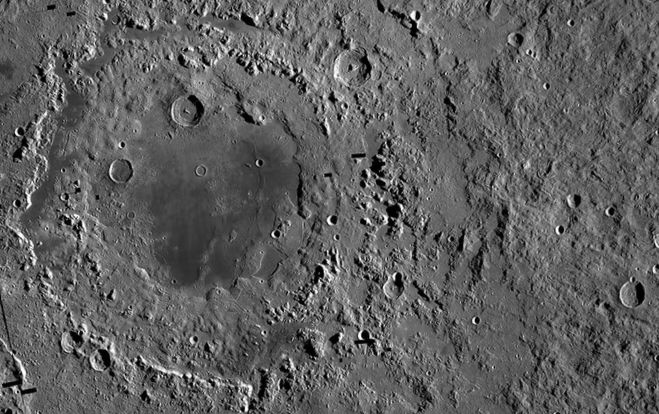
The chains of holes and the squiggly valley segments are interpreted as the beginnings of collapse of the empty lava tunnel, long after the molten lava had stopped flowing through it. The long, open, squiggly valley represents the stage when the entire lava tunnel roof has collapsed. Where was this lava flowing to? Schröter’s Rille† is probably the easiest squiggly valley for amateur telescopes, and it shows that lava was flowing from a point of eruption in the Highlands toward the floor of the western part of the Mare Imbrium. This represents good observational evidence that the dark material covering the Mare floors is indeed lava, and you can see it for yourselves!
The most famous of all lunar valleys is probably the Alpine Valley that cuts across the Alps on the northeastern side of the Mare Imbrium. Most people think of it as a straight valley and interpret it as a rift, but if you looked very carefully at a high-resolution photo, you would see that there is a squiggly lava tunnel running all the way down the length of the rift. Although the Alpine Valley is very easy to see when the shadows are just right, I don’t expect to be able to see the lava tunnel with any amateur telescope. But I may be wrong, so let us know if you do! Scarps and Ridges Scarps are steep slopes that form when a large fault reaches the lunar surface and the ground on one side drops down with respect to the other side of the fault.
The most famous is perhaps is the Altai Scarp that runs parallel to, and faces toward, the Mare Nectaris. It represents a segment of the circular ridges created by the original impact that formed the Nectaris Basin. This scarp is gigantic, rising to an average of 1.5 kilometers high, and locally up to 4 kilometers high! Another very well known example is the “Straight Wall”† that faces toward the Mare Nubium. However, because it is so straight, it is probably a late fault scarp that formed long after lava filled the original impact basin. Selected Craters and Features Listed on many maps as “Rupes Recta” Editor Ridges are rather like low, rounded scarps that occur in the margins of Mare. Traditionally, they are interpreted as scarps related to the original impact basins that have been covered by the Mare lavas, and in many cases this is probably true. But, instead of being continuous, ridges in the southeast corners of the Mare Humorum and Mare Serenitatis seem to be made of offset segments, and there are several small craters that lie along the crests of some of the ridges.
Also, if the light is right, you can see that the ridges are either symmetrical, or they were scarps that faced towards the exterior of the Mare. But, scarps related to impact basins should face inwards towards the excavated hole! These ridges look to me like late faults that have channeled lava from deep down; molten rock that has reached the surface as volcanic craters located along the fault network. I think these fault ridges are younger than the Mare lavas, but old enough for volcanic activity to still have been possible.
While you might see the ridges through your telescopes, I doubt you will be able to see these small craters. Maybe this is a lunar observing challenge? Timelines Light colored linear streaks radiating from some impact craters can extend halfway round the Moon. These crater rays represent debris ejected from the craters at the moment of meteorite impact. Any landforms that are crossed by the crater rays must be older than the crater that the rays originated from.
Two of the best preserved examples are related to the Copernicus and Tycho craters. Copernicus formed about 1 billion years ago, at about the same time as the rocks that make up much of Southern Ontario, including the Foymount observing site south of Renfrew and the FLO. Crater rays from Tycho cross all of the Mare on the lunar near-side, demonstrating that Tycho is the youngest large impact crater on the Moon’s surface. It formed about 100 million years ago, when the dinosaurs were still in their prime. Make the most the Moon’s proximity to Earth to directly observe for yourselves the evidence for its geological history, and bear in mind that you’ll be observing the scars left by phenomena very similar to those that have shaped the other rocky planets of our inner Solar System.
Lunar Cataclysm the Late Heavy Bombardment
The Moon does reflect major bombardment early in the history of the Solar System, but do we really know what that bombardment represents, how and when it evolved, and what consequences it might have for understanding how our Solar System, our own planet and even the life that covers it evolved? With these questions in mind, let’s have a look at what we know of this Late Heavy Bombardment and how we know it.
The Late Heavy Bombardment is an integral part of the accepted accretionary history of our Solar System as a planetary system.
The standard model for accretion traces the progressive formation of clumps of dust grains that accrete to form km- to 100km-size planetissimals, that accrete to form protoplanets in the 100-1000 km size range. Given this context, how does the Late Heavy Bombardment fit into the picture?
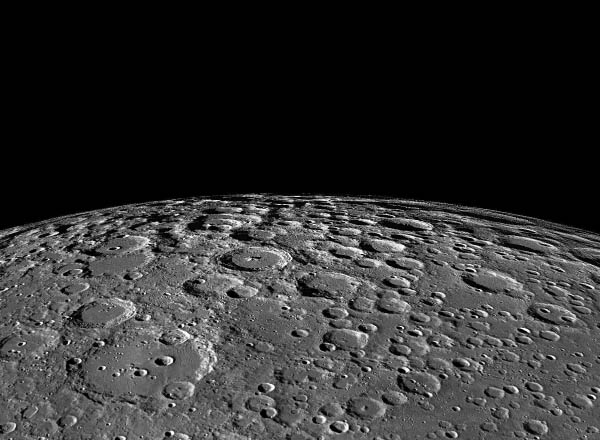
Before we address that question, it’s just as well to remind ourselves that until the early 1960s scientists were still arguing as to whether lunar craters and basins were of volcanic or impact origin. Resolving this debate owed much to the early work of Bill Hartmann, shown here working on a pre-Apollo Rectified Photo Atlas in order to simulate orthographic or “overhead” views of craters and basins by pasting images on a 3D globe and re-photographing them to correct for the distortion due to the foreshortened view of the edges of the lunar disk as seen by Earth-based telescopes.
Having established that lunar craters and basins are indeed impact features, the next step was to quantitatively calibrate the history of bombardment through absolute time. NASA sent men to the Moon For security reasons, all of the Apollo samples come from near the equator on the lunar near-side, from sites fairly close together compared with the total surface area of the Moon. The favoured samples for dating the lunar impact history were ejecta materials preserved as welded rock fragments (impact breccia) and impact melt.
The initial work focused on the analysis of radioactive U (uranium) and its ultimate breakdown product Pb (lead) in zircon grains in impact breccia samples. Sure enough, there seemed to be a peak in ages at ~3.9-3.8 Ga that was attributed to the disturbance of the U/Pb clocks in zircon grains by the heat of formation of the major Impact Basins. BTW Zircon is a form of zirconium silicate ZrSiO4. However, it turns out that this approach may have been a little too simple, and the consensus view may warrant a new perspective.
Let’s take a look at an example of this “new perspective”. Work on terrestrial impact features in South Africa, published in 2015, demonstrated that zircon grains in shocked impact breccias do not always record the impact event. Most often they record their original crystallisation from magmatic melts, well before impact. Now, it is possible with very careful selection of which part of the zircon grain one analyses to separate the time of crystallisation from the time of impact. But, that’s the problem: the early work on Apollo lunar material was not done using this approach. All of which means, we’re not sure of the significance of the ~3.9-3.8 Ga ages derived to date.
From a different point of view, planetary scientists have been asking for sometime whether the consistent ~3.9-3.8 Ga ages are an indication that one of the youngest major impacts Imbrium is the usual suspect may have coated the near-side of the Moon with its ejecta, thereby swamping earlier ejecta from other impact basins. When you put these two ideas together, they could mean that ~3.9-3.8 Ga on the lunar near-side is actually the age of the rocks that got pulverised during the Imbrium impact, whenever that occurred.
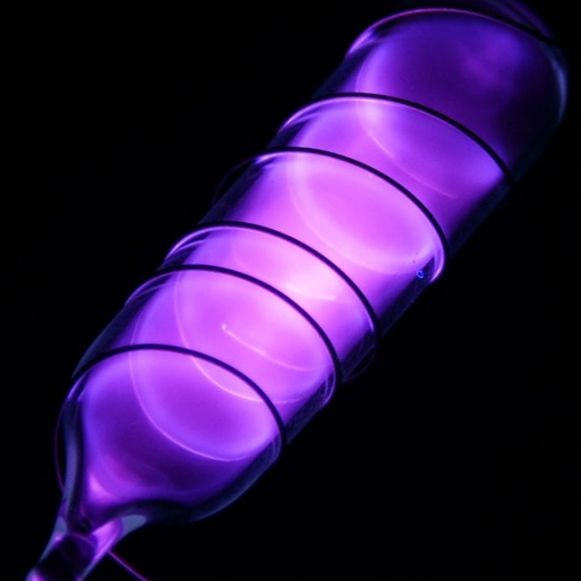
Well, so much for U/Pb in zircon grains in impact breccias! Is there something else we can use to date impacts on the Moon? Yes there is isotopes of Ar (Argon). The general idea with Ar is that if you analyse it in the impact melt, it will record the age of the quenching of the impact melt, and thereby the time of the impact itself hence getting around the issue of having to interpret the significance of the zircon ages. This diagram of the number of Ar ages from Apollo samples of the lunar surface through time does indeed seem to confirm the existence of a discrete peak of impacts at about the time of the purported Late Heavy Bombardment ~3.9-3.8 Ga. But, it’s not that simple. Ar isotopes can also be reset by the heat of impacts. In other words, a sample attributed to a given impact “A” may actually record a resetting of the Ar clock by a younger impact “B”, which brings us full circle and back to square one.
Using similar reasoning as for the U/Pb ages from zircon grains, we are faced with similar questions do the Ar ages from lunar impact melts record a global bombardment event across the whole Moon, or do they just record impact melt ejected from the Imbrium Basin impact event?
In fact, you could ask if the impact melts and the impact basins they are spatially associated with are in fact even older than ~3.9-3.8 Ga, and were simply re-set by something major at ~3.9-3.8 Ga. In other words, was the formation of lunar impact basins tied to a Late Heavy Bombardment that occurred earlier than ~4.0 Ga and if so what does the major resetting of ages at ~3.9-3.8 Ga represent? Whatever none of this helps us to unequivocally determine the age of formation of any given impact feature (basin or crater), and leaves us all very frustrated (lunar scientists), if not confused (amateur astronomers). But, for now, let’s continue with the consensus view that says that U/Pb and Ar ages of ~3.9-3.8 Ga on the Moon do indeed reflect the Late Heavy Bombardment also referred to as the Lunar Cataclysm hence the title of this presentation.
Why would the Late Heavy Bombardment occur some ~700 Ma or more after the main accretionary events that formed the planets of our Solar System? There’s no consensus on this question, but there is a leading contender: the Grand Tack or Nice model of planetary migration that I’ve discussed with you before in my article Is our Solar System and the Earth within it “Normal”?. Put simply, it goes like this: before the Rocky Planets (Mercury, Venus, Earth Mars) formed by accretion, Jupiter and Saturn migrated inward toward the Sun. Then, due to gravitational interactions (orbital resonances), they turned around and migrated back out again. It took over 500 Ma for the outward migration to push Neptune and Uranus into and thoroughly disturb the Kuiper Belt of comets and asteroids that sits outside of the planets, which catalysed the migration of many Kuiper Belt objects into the inner part or planetary domain of the Solar System, where they gave rise to the Late Heavy Bombardment as a discrete event.
The initial planetary accretion ending very quickly after 4.5 Ga, and the Late Heavy Bombardment occurs as a sharp spike that disturbs an otherwise relatively calm Solar System. But other models proposed by other scientists yellow and blue lines give us a very different picture. What if the rate of accretion and impacts decreased much more progressively? What if the Late Heavy Bombardment was indeed late, but not very heavy; maybe even just a subtle uptick in impact rates? Well, let’s look at some data and see what they say.
Take a look at the diagram of impact resetting of age dates using Ar instead of U/Pb. The red line as we’ve already seen in slide #10 is for Apollo samples from the Moon, and it shows a clear spike at around ~3.9-3.8 Ga, that could correlate with the proposed Late Heavy Bombardment. But, now look at the distribution of ages recorded by meteorites (black and blue lines) including lunar meteorites (green line). They show much wider spreads of impact activity, with nothing particularly special happening at the 3.9-3.8 Ga mark. In other words, we only see the Late Heavy Bombardment as a major event localised in time at ~3.9-3.8 Ga when we look at Apollo samples from the near-side of the Moon. In a broader sample of ages from elsewhere in the Solar System and other parts of the Moon the Late Heavy Bombardment is not so apparent.
Why is this discussion of the purported Lunar Cataclysm so important? Because it has implications for the overall evolution of the Solar System as a whole, our understanding of the formation and evolution of planetary systems in general, and the evolution of the Earth and life as we know it! In 2009, a Community White Paper concerning the Moon prepared by a slate of planetary scientists of international standing stated that we are currently unable to distinguish a progressively decreasing impact history from one that includes a Late Heavy Bombardment event at ~3.9-3.8 Ga. What are the repercussions of such an admission? Let’s look at just 3 of them.
First, our understanding of the absolute ages of surfaces on planets other than the Earth is entirely based upon the premise that we can extrapolate the time calibration of the bombardment history of the Moon to other worlds within our Solar System. This premise has long been questioned: why should the bombardment history of the inner Solar System be the same as for the outer Solar System? What about the gravitational effects of the giant planets: wouldn’t they attract more impactors than the smaller rocky planets? However, without such a premise, we couldn’t even start to compare and contrast the bombardment and resurfacing histories from one planetary body or moon to another. Yes, we could still say that this part of a planetary surface is older or younger than another on the same planet or moon, but we would have no absolute age yardstick. This would mean that we could not formulate a robust history of events across the Solar System.
Second, the leading model for the formation and evolution of the Solar System as a whole the planetary migration model I briefly summarised earlier in slide #11 is entirely predicated on accounting for the existence of a sudden, ephemeral outburst of impact bombardment at ~3.9-3.8 Ga. If the Late Heavy Bombardment never happened, if it is an illusion, then we go back to square one in trying to explain why our Solar System looks like it does. This is a serious, very serious, scientific conundrum.
Craters Meton and Pitatus
Craters Meton and Pitatus are a wonderful study in contrasts. Both are categorized as flooded walled plains that is, craters greater than about 70 km in diameter that have been flooded with lava or ejecta but they present wildly different views to an earthbound observer, especially under drastically different lunar lighting conditions. So let’s get started.
Not far from the north pole of the Moon lies a 122-km system of interconnected flooded craters known as Meton (73.8º N, 19.2 E; Rükl charts 4, 5). Named after a 5th century Greek astronomer/mathematician, Meton is among the oldest craters on the Moon, predating the giant Mare Imbrium impact of 3.8 billion years ago. The crater and its sisters are filled with ejecta from that impact.
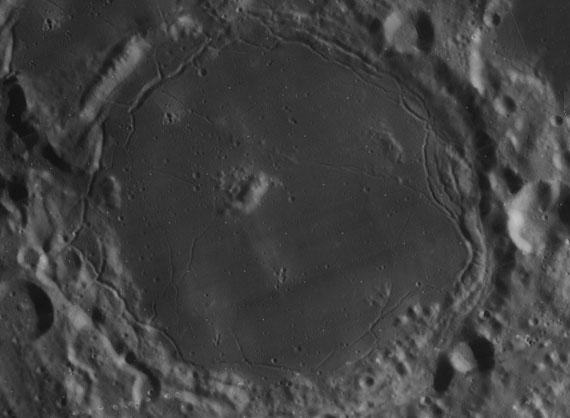
From Earth, the crater along with its large constituents Meton C and D appears to be much longer east to west than it does from north to south. But this is only because of its position so close to the northern limb of the Moon. Spacecraft images show a rounder Meton that is much more equally proportioned. When I observed and sketched Meton at first quarter on March 28, that sector of the Moon was librated slightly away, making the already low viewing angle even more oblique.
Crater Pitatus, with its pulverized, two-kilometre-high walls, is located on the southern shore of Mare Nubium, the 700-km-wide Sea of Clouds. The impact basin underlying the 500-metre-deep lava plain of Mare Nubium is one of the oldest on the Moon, dating back 4.2 billion years. Lava-filled Pitatus and a companion crater Hesiodus (43 km) adjoining it to the west appear to be battered relics of that ancient past.
Under the flat lighting, both craters showed a remarkable patchwork of subtle tonal differences. The darkest areas were found in Pitatus in a line cutting north-south along the western side of Pitatus’ off-centre peak, and in a thick band across its southern floor. A broad ray from Tycho 250 km to the south passes not too far to the west of these craters in very dramatic fashion, like some kind of grand avenue. The overall effect of this strangely lit moonscape is, oddly, that of a thriving civilization.
Total Lunar Eclipse
A total lunar eclipse is the result of the Earth’s shadow crossing the moon’s disk. If you think about this, it’s clear that, for the Sun to be throwing the Earth’s shadow directly at the moon, the Sun and Moon must be on exactly opposite sides of the Earth. That’s also the definition of Full Moon, which is why total lunar eclipses can only occur when the moon is full. (Why isn’t there an eclipse every time the moon is full? That’s discussed below.)
Umbra Penumbra
The area of complete shadow behind the Earth is called the Umbra. Anyone in the Umbra would not be able to see any part of the Sun.
In the Penumbra area, part of the Sun will be obscured by the Earth, but part will be visible. For example, someone near the word “Penumbra” in the diagram above can see the upper part of the Sun but not the lower part.
We say the moon is in total eclipse when it is entirely in the Umbra in complete shadow. When the moon is in the Penumbra it is, technically, receiving less sunlight, but the Sun is so bright that the difference is not noticeable to a casual observer.
Why isn’t it Invisible? Why is it Red?
When the moon is in total eclipse, it is in the Umbra, which means it is in complete shadow. So why can we still see it? A moon in total eclipse doesn’t disappear, it just turns a very dark smoky red colour.
The moon appears red for the same reason that sunsets on the Earth appear red. The earth’s atmosphere contains particles (dust and moisture) that have the effect of scattering the light that passes through it. Red light is scattered the most (the amount of scattering is a function of the wavelength of the light being scattered as related to the size of the interfering particles).
As sunlight streams by the Earth, the thin layer of atmosphere scatters some of the red light, throwing it into the Umbra. So objects that should be in full shadow are, instead, received a small amount of red-coloured illumination from scattered sunlight
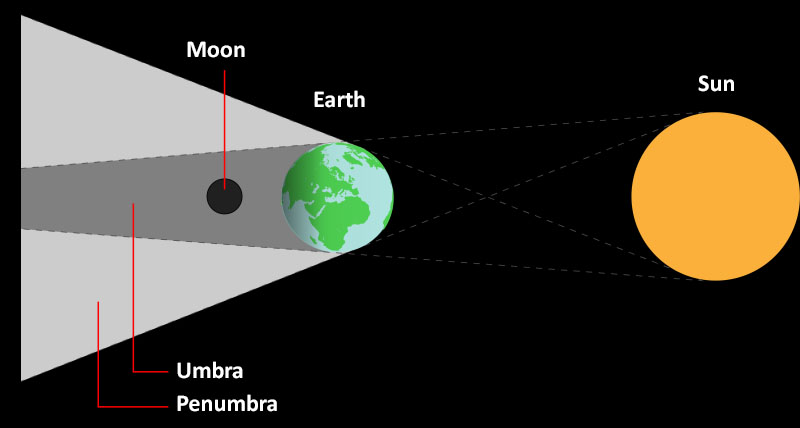
When do Eclipses Happen?
As mentioned above, a total lunar eclipse requires a full moon, but a full moon does not guarantee a total lunar eclipse.
That’s because the plane of the moon’s orbit around the Earth is not exactly in line with the plane of the Earth’s orbit around the Sun. If these were precisely aligned, then every time there was a full moon there would be a total lunar eclipse.
Instead, the plane of the moon’s orbit around the Earth is tilted about 5 degrees from the plane of the Earth’s orbit around the sun.
The result is that the moon’s orbit frequently places it outside the Earth’s Umbra. Only when the tilted orbit happens to bring the moon in line with the Earth-Sun line does a total eclipse occur.
There are always at least 2 total lunar eclipses in a year, and there can be as many as 3. On average, there are 2.44 total lunar eclipses per year.
Lunar eclipse geometry
Lunar eclipse geometry is quite different from that of a solar eclipse. In much the same way as a doorframe casts deep and faint shadows, the Earth casts a dark, central umbra surrounded by fainter penumbra. When the Moon doesn’t enter the umbra at all, the eclipse is called penumbral. A total eclipse occurs when the Moon completely enters the umbra. A partial eclipse occurs when the Moon does not completely enter the umbra. Penumbral eclipses and eclipse phases are subtle events. It usually takes a careful observer to notice the penumbral shadow moving over the Moon. For this month’s eclipse, the first penumbral phase starts shortly after sunset, making it even harder to notice the faint shadow. Once the moon starts to slip into umbra, at about 10 pm, the eclipse starts to get interesting. As the eclipse moves towards totality, the deep umbral shadow slowly creeps past craters and other landscape features on the lunar surface. When the Moon begins to emerge from the umbra, the shadow boundary unmasks a different set of lunar features, so there are new observations to make after totality.
The reddish color of a picture-perfect eclipse is caused by sunlight filtered and diffused through the Earth’s atmosphere. There are so many variables that it’s difficult to predict in advance how the Moon will appear during the 52 minutes of totality. The eclipsed Moon can range in color from nearly jet black to a bright orange-red, and the shade can vary throughout the umbral phases. The Danjon scale is commonly used to rate the depth of the eclipse shadow.
Photographing a lunar eclipse is a straightforward project. You can get surprisingly good results with even a tripod mounted camera. Any of the more advanced astrophotography techniques can produce incredible photographs. One challenging technique for recording the progress of the eclipse is through in-camera multiple exposures. It’s a lot less nerve-wracking to take multiple frames and stack them with photoediting software.
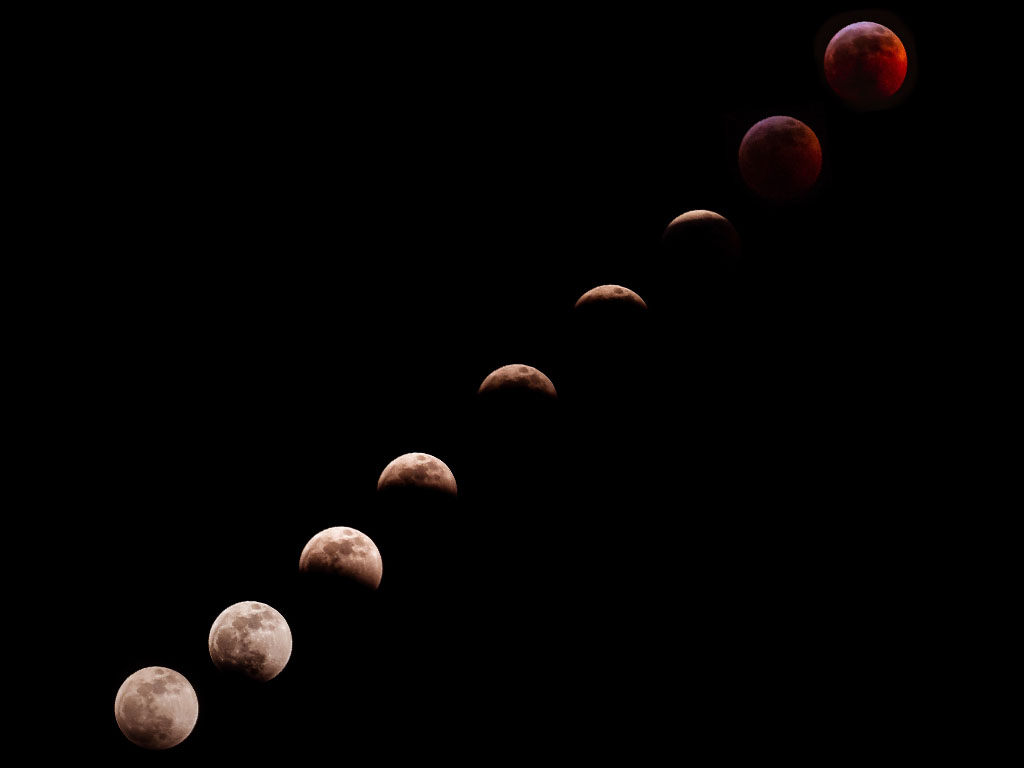
In Ottawa, one can drive out 20 or 30 km in almost any direction to find reasonably dark skies. For the more adventurous, there are excellent observing sites just a few hours away.
Here are a few of my picks:
The Echo Valley Observatory, Muskoka The Echo Valley Astronomical Observatory is Canada’s first resort-based astronomical observatory and the largest in Ontario to be used primarily for public viewing of celestial objects. In the words of Terence Dickinson, Canada’s well _known astronomer: “This new observatory offers the visitor a complete astronomy experience unlike anything previously available in Ontario. It is located far enough from city lights that the beauty of the universe is wonderfully accessible.”
Situated at one of the highest points in Muskoka, about 12 kilometers east of Huntsville, the three-story domed Echo Valley observatory houses a state-of-the-art 40 centimeter Schmidt-Cassegrain telescope. The area is renowned for its dark skies free from light pollution. On a clear, dark night, these are some of the sights that you can expect to see Ammonia cloudbelts of Jupiter and 4 of its moons Rings of Saturn and half a dozen of its moons The Orion Nebula in all its glory, and many other deep sky objects Just a 400 km drive from Ottawa, Muskoka is a also a fun filled holiday destination for the entire family.
This is the ultimate destination for the dedicated amateur astronomer, housing the largest telescope on the East coast in the midst of the idyllic Parc National du Mont Megantic, situated in the Eastern Townships of Quebec, about 450 km from Ottawa. Mont Mégantic’s modern-day existence dates back to the 1960s when Montreal University recognized that the summit of Mont Mégantic would be an ideal site for astronomical observation because of its elevation and its clear, pollution-free skies. The University leased 10 hectares of land from the Quebec government to build the Observatory. It began operating in the spring of 1978. In fact, the observatory inspired the Park’s theme “From the earth to the stars”. Astronomy activities for visitors begin with the Astrolabe, at the base of Mont Megantic with the Observatory’s 36″ telescope. With dark skies all around the visibility is exceptional on clear nights. The visitors then proceed to the summit, and if the night is clear, they get an opportunity to look through the 61″ telescope. If the clouds prevail, the multimedia observatory provides unforgettable interactive virtual sky observation. The observatory opens to the public around the middle of May.
It has the darkest possible skies within a few hours drive of Ottawa. Seventh magnitude stars are readily visible to the naked eye on a good night, while the Milky Way glistens like a jewel in the clear night sky. The main observing site is an abandoned airstrip but permission from the Park authorities is required prior to using it. No camping is allowed at the airstrip itself, but excellent campsites are available just a few kilometers away.
Asymmetrical Moon
Let’s start with a simple observation: not only is the Moon asymmetrical on the surface, but its centre of mass is not at the centre of its shape. Geologically, the reason for the offset of the centre of gravity is because the light-weight crust, or the outer layer of the Moon, is thicker on the far-side than it is on the near-side, while the inner layers, called the mantle and the core, appear to be concentric, like the stone in a peach. Generally available planetary geology texts1 appear to attribute this difference in crustal thickness to the gravitational influence of the Earth, potentially relating it to the fact that the Moon always presents the same face to us here on Earth.
When satellites such Lunar Orbiter and Clementine systematically orbited the Moon, the different crustal thicknesses influenced the Moon’s gravity field, tugging on the satellites as they passed over different parts of the lunar surface. By measuring the effects of gravity on the satellite orbits, planetary geologists can calculate models for the thickness distribution of the lunar crust. What all this means is that if a magma or lava is produced at a given level within the lunar interior (i.e. the mantle), it has further to travel in order to reach the surface on the far side, compared with the near side. Lava cools as it rises, so there’s a greater chance that the lava will freeze before it gets to the surface on the far side. Therefore, there are more lunar Mare on the near side.
Magma Buoyancy
Actually, its just a little more complicated than that! Bubbles and hot-air balloons rise because they are lighter than the stuff they are rising through, whether it’s air or water, cooking oil or custard! In part, lava rises for the same reason: because it’s lighter than the stuff it’s moving through. And the reason it’s lighter is because it’s hotter!
The lunar crust is about 70 km thick on the far side. Most importantly, it is denser in the lower part and lighter in the upper part. When we say that the lunar crust is thinner on the near side, this means that much of the upper lighter part is missing. Therefore, lavas only have to rise through the lower, denser part to get very close to the lunar surface. In contrast, on the far side, the same lava has to rise through a good thickness of light crust before it reaches the surface. If the lava is not hot enough, it will be too heavy to rise through light lunar crust, so it gets trapped underground where it freezes and stops moving. What all this means is that Mare lavas reached the surface of the Moon on the near side much more easily than on the far side.